These are my cleaned-up day by day notes from a 13 day geology field trip on the big island of Hawaii to explore the volcanic features of the island.
Day 1 (1/24): Sturgeon Lake; Pahoehoe; Hydrothermal Alteration
Sturgeon Lake Deposit (George’s thesis)
George talked about his study of the Sturgeon Lake area – he mapped 20 x 5 miles. His interpretation is that there were two eruptions. The first was a huge subaerial eruption at 2.7 Ga (neo-Archaean) – it was the size of the Yellowstone volcano, and produced at caldera 25 Km in diameter. Most of the material from crater exploded into the air. After the first eruption the caldera collapsed and was filled with water; possibly sea level also rose or the land subsided. A subsequent subaqueous eruption created a 5 Km caldera. Unlike the previous eruption, this one would have been capped, so that the material was retained in the caldera, including sulfides that would have degassed if it were subaerial – this led to the creation of sulfide deposits.
If you do the back calculation, there’s a really nice correlation between the diameter of the Caldera and the eruption volume.
Of volcanic eruptions Fischer said:
Igneous on the way up, sedimentary on the way down. — Fischer
About pahoehoe: flows, channels, olivine…
- Tube-fed flows produce both pillows and pahoehoe.
- Pahoehoe can be seen on the North side of St Luke’s hospital in Duluth on 1st St.
- Pahoehoe cools AND loses gas which increases viscosity, which can further the tranisition to a’a.
- Pahoehoe flows, a’a’ rolls
- Facies: Close to the vent you’ll see blisters; then you get slabby pahoehoe; then ropey — because interior flow rate has slowed and lava is ductile.
- There are great pictures of pahoehoe in Day 2.
We visited a road-cut that featured an infilled lava channel, with columnar jointing and surface oxidation.
At stop 7 we saw very thin pahoehoe flows. Olivine rich at top, olivine poor at bottom. You can see the tubular structure of the pahoehoe flows.
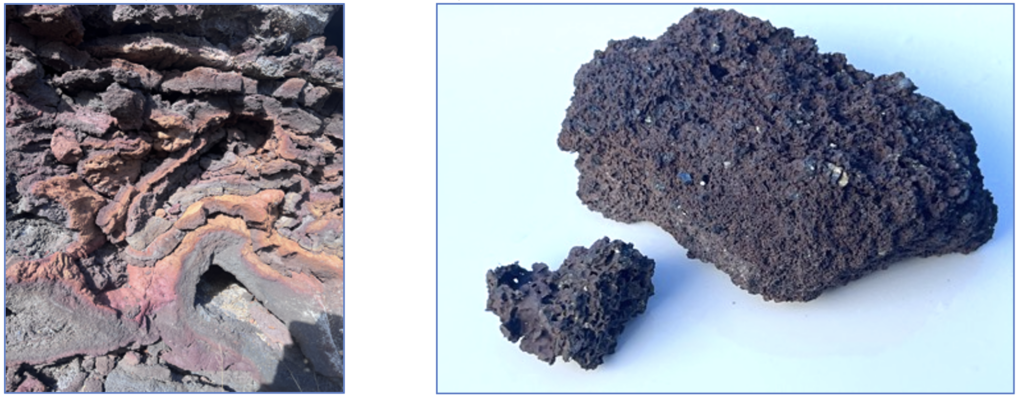
Notes on hydrothermal alteration
(cf. Kilauea caldera steam vents)
Steam is altering the basalt. This is metasomatism — hydrothermal alteration — where the minerals’ compositions are altered i.e. the chemicals present in them are changed due to the hydrothermal fluids and gasses.
The white stuff is “sinter:” The most common form of sinter is silica sinter, which is made primarily of silica (SiO₂), though it can also contain other minerals depending on the composition of the geothermal fluids. There is also some calcite. This system is near neutral — elsewhere you’ll have more acidic. That will produce phosphate and sulfide minerals. These are often implicated in ore formation.
We are seeing steam-heated overprinting that is producing kaolinite. In geological terms, an “overprint” refers to a secondary mineralization or alteration event that affects a pre-existing rock or mineral deposit. In this context, it means that steam is modifying the plagioclase in basalt to kaolinite. This is one reason that in figuring out the geological history of an area you have to map – the composition of the rock is not enough.
Hydrothermal systems associated with ring faults are self- reinforcing systems. The alteration weakens and exacerbates the faults, which in turn creates or widens channels that allow steam to emerge.
Day 2 (1/25): Kilauea fountains & stratigraphy
The First Eruption
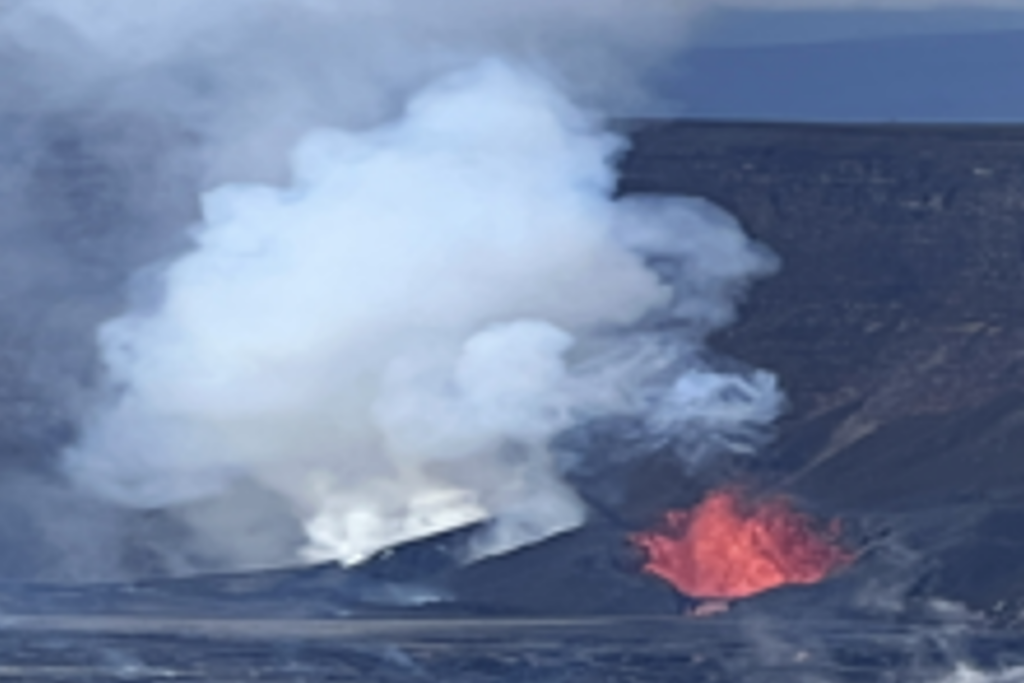
At the Kalauea caldera we arrived to find an eruption in progress. We are seeing lava fountaining and lots of smoke. The column of smoke was amazing to see – quite beautiful! The geologists are reading the (slight) colors in the plume and inferring which volatiles are degassing.
Pele’s hair (sideromelane). There is golden Pele’s hair on the ground. Pele’s Hair is made of a glass called sideromelane. Golden Pele’s Hair was quenched at a high temperature before iron oxice crytlas could form; brown Pele’s Hair cooled more slowly, allowing time for some crystallization. In the presence of water, Pele’s Hairwill alter to palagonite.
Someone brought (and shared) binoculars, which provided a very nice view of the rift, lava lake, pahoehoe flows, and other features.
NOTE: Caldera Collapse does not occur AFTER the Eruption — rather eruption and collapse are synchronous. Ancient caldera collapses are indicated by mega-breccia.
Base Surge Stratigraphy: crossbedding lapilli, bombs, and ripples
The penultimate stop was also excellent. We went along the closed section of Crater Rim Road (starting from the Desolation Trailhead). This offered another view of the eruption. There was also a spatter rampart, built of glossy glassy blobs of lava – I think the name of this rock/texture was “agglomerate.”
Right at the point where the road is blocked off there are ash layers, etc., produced by a base surge (a pyroclastic eruption which projects materials in all directions). More of these features will be visible in the Ka’u desert hike on Day 3.
It was interesting to see how well the geologists could ‘read’ the layering. We saw crossbedding (right), and (below) accretionary lapilli, bombs and ripples.
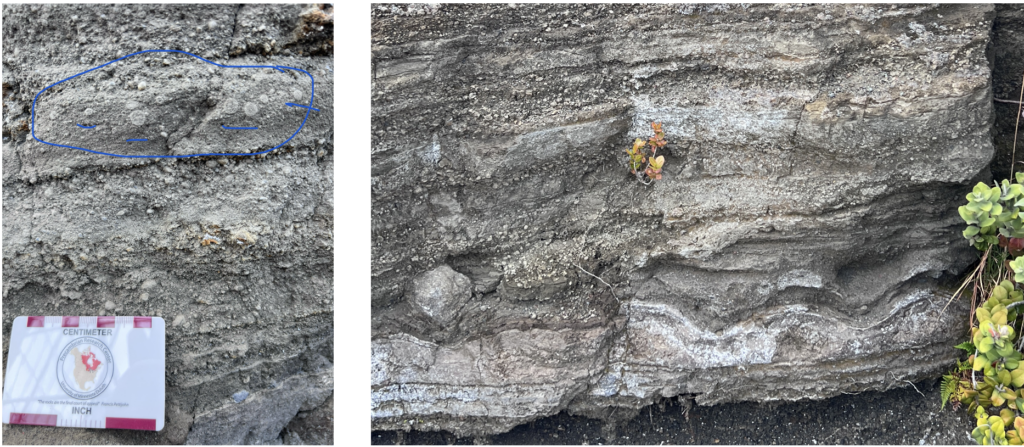
Base Surge Facies
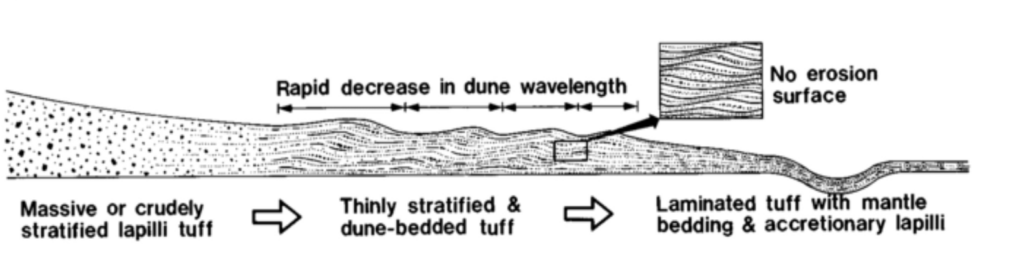
Day 3 (1/26): Kau Desert: A’a, pahoehoe, Pele’s Hair, Ash and Lapilli
The hike starts out in an a’a lava field and then transitions to a different flow that is largely pahoehoe. This area has really beautiful examples of ropey pahoehoe.\
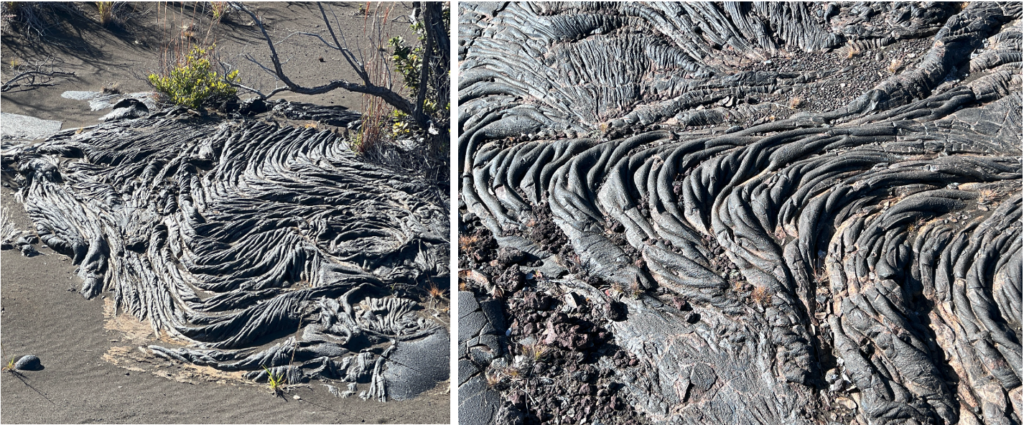
Two other images show the imprints of spatters of lava drops, and tortoise shell cracking. …and one accretionary lava ball from the a’a section of the hike because I had room…

We also saw great examples of ash layering, ventilation effects (wind from base surge blowing across wet ash), and pele’s hair.
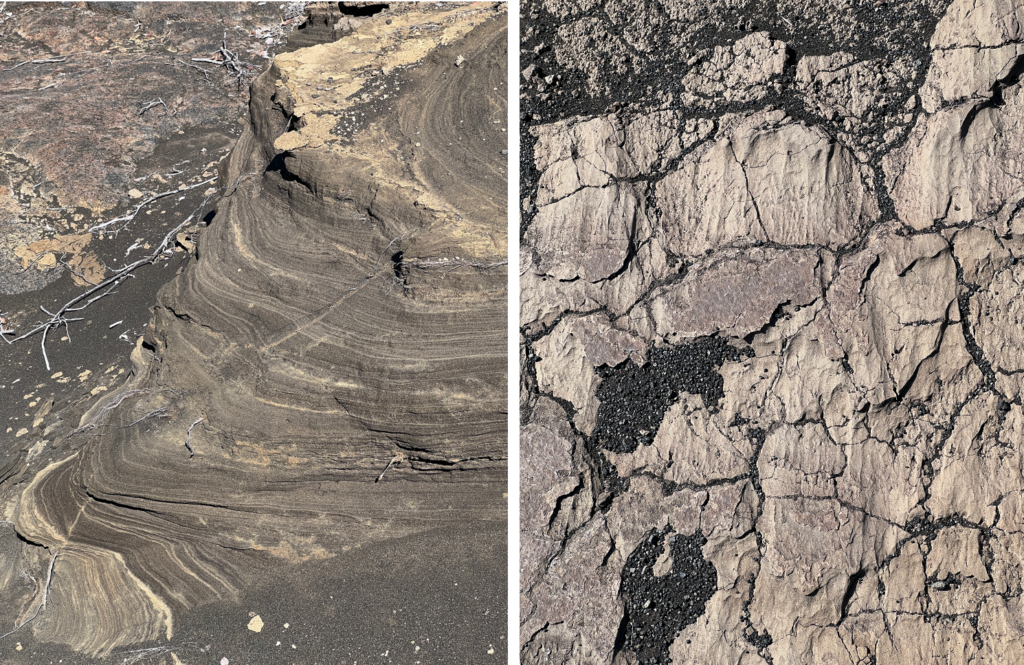
We also saw lapilli, and I learned that lapilli refers only to size; only (some) accretionary lapilli are spherical and composed of concentric shells.
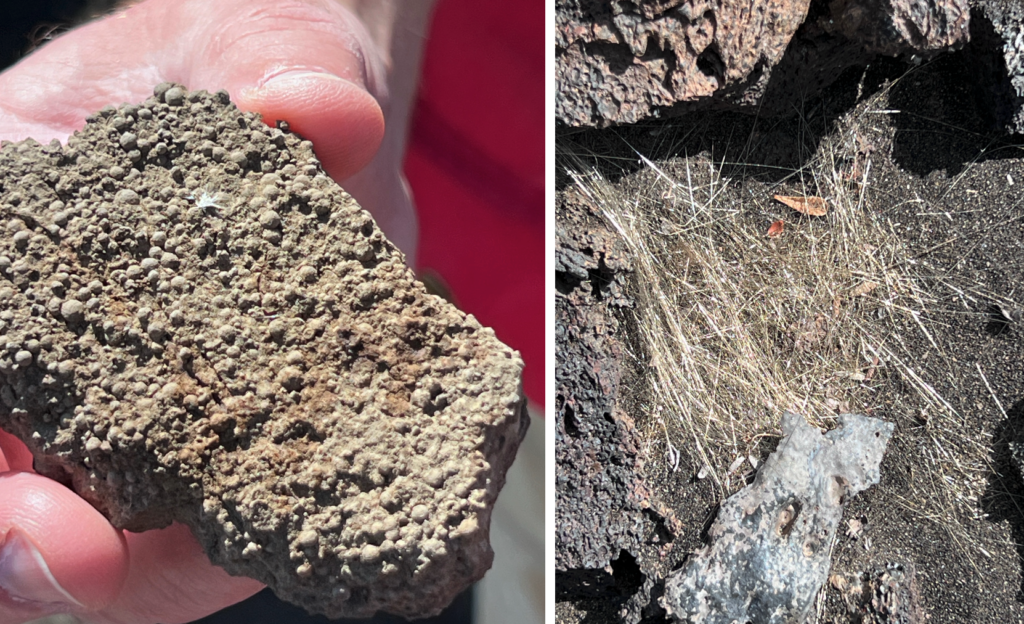
I was dismayed that, by the end of this hike which was no more than 5-6 miles, I was feeling a lot of fatigue.
Day 4 (1/27): Lava field with lava trees; and a spatter rampart
Lava field, with trees
The day began with a drive down Chain of Craters road. Our first stop was at a site near Devastation Trailhead, next to a pit crater, that had lava trees – that is, lava had flowed around tree trunks and cooled against their surfaces, forming blocky pillars, often with a cast of the trunk inside (and, occasionally, with some of the tree remaining). This flow happened in 1974.
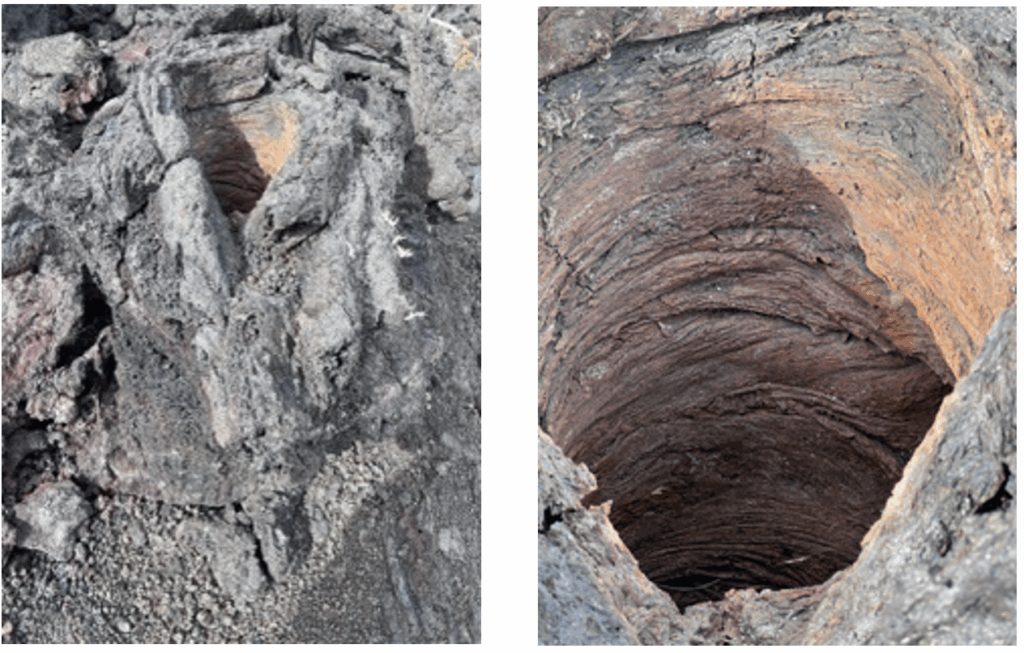
We had to go about an eighth of a mile to find the lava trees, and as we did so we found that we could see that this was a very small lava field, and that we could see all of its features. We found the vent from which the eruption began along with a spatter rampart (whose asymmetry shows the direction of the wind during the eruption), the slabby pahoehoe that is characteristic of near-vent flow, and the main lava channel that drained the field into a nearby pit crater in a lava fall.
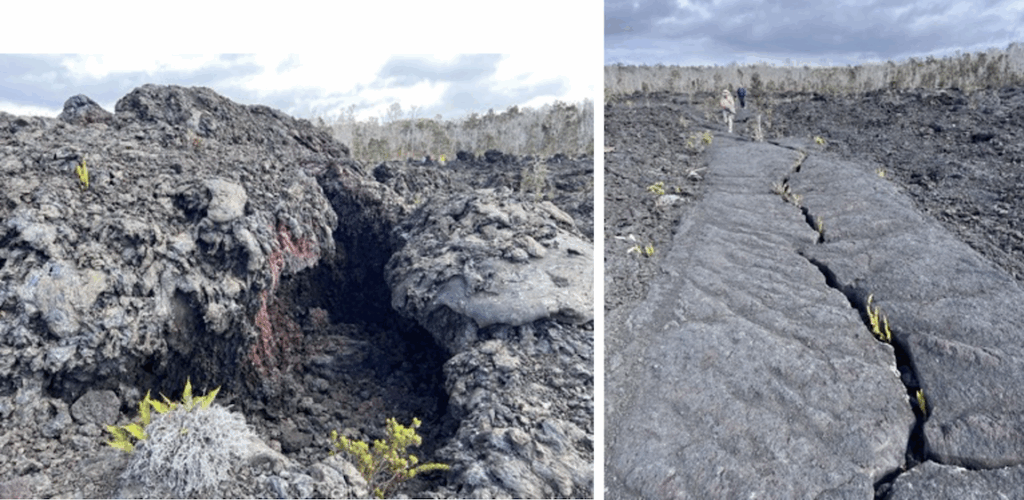
Rift, with spatter rampart
Our second stop was Napau Trailhead, which provides an excellent look at a rift, with spatter ramparts and drainback features. This spatter rampart was formed in about a day.
Rift (with vegetation), and spatter ramparts to its left (indicating a strong wind blowing in that direction):
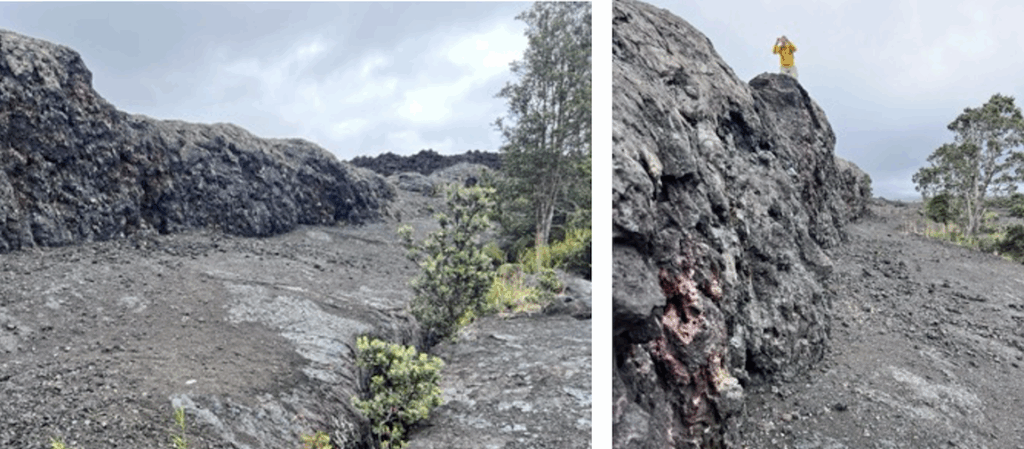
Spatter ramparts are cool because they have lovely textures from the agglomeration of spatter, which often oxidizes into reds and orange colors, and has glassy surfaces because of rapid cooling.
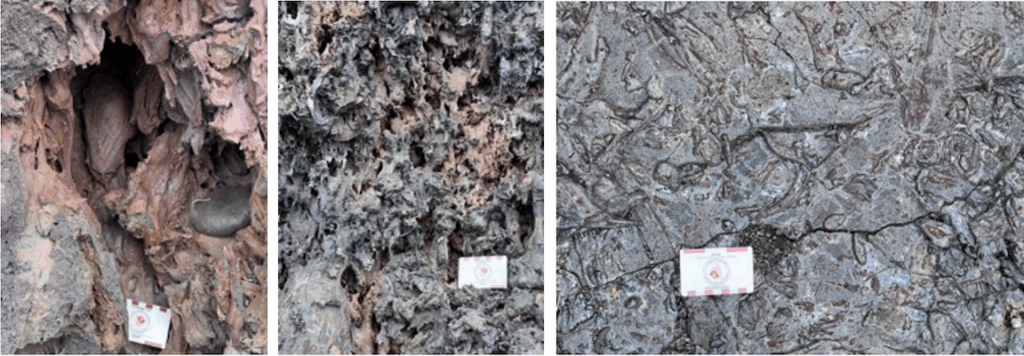
As the eruption abates, the lava subsides back into the rift, leaving what are called drain-back features:
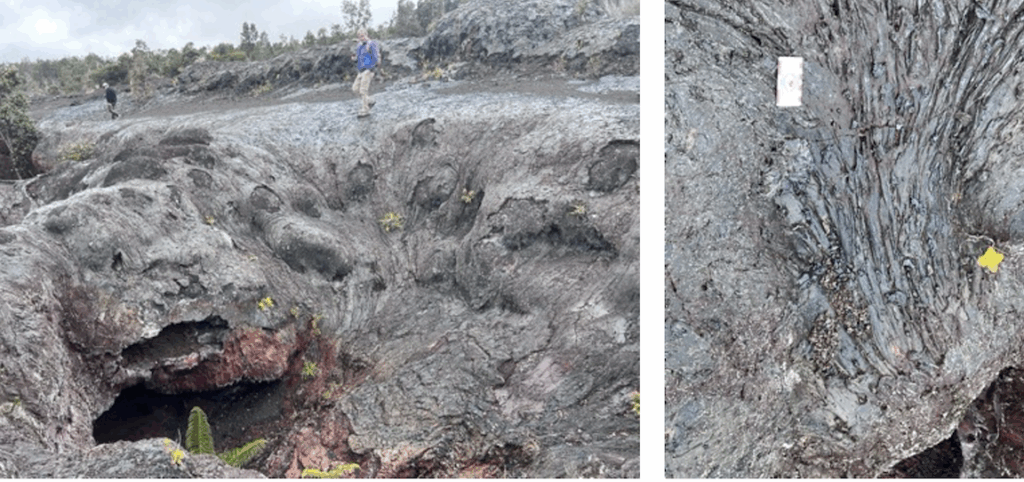
The protrusion below is likely a deflation feature, left behind as the level of lava dropped through draining and shrunk, through cooling. (Al originally thought these were hornitos, but George the volcanologist did not agree).
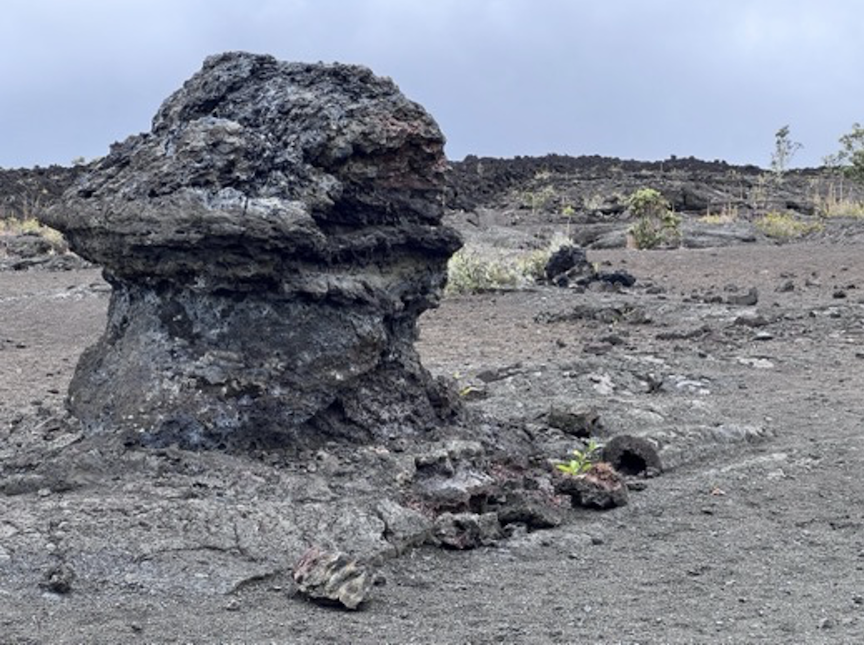
Spatter rampart terminology: Juvenile fragment == spatter; accessory fragment == a solid ejected from the rift; accidental fragment == anything not associated with the source of the lava such as a xenolith or local rock.
A second episode of the eruption
At the end of the day, we had dinner in the bar at Volcano house. During dinner the eruption started again. At first all you could see was smoke reflecting an orange glow below, then there was a small bright spot that pulsed about once a second. Overtime the spot grew into a long curtain-like fountain that grew higher and higher. As this happened you could see a glow spreading out from its base, a growing lake of lava. As the fountain increased in size, the erupting lava fell on the slope from which it is erupting, and flowed rapidly downhill in three streams.
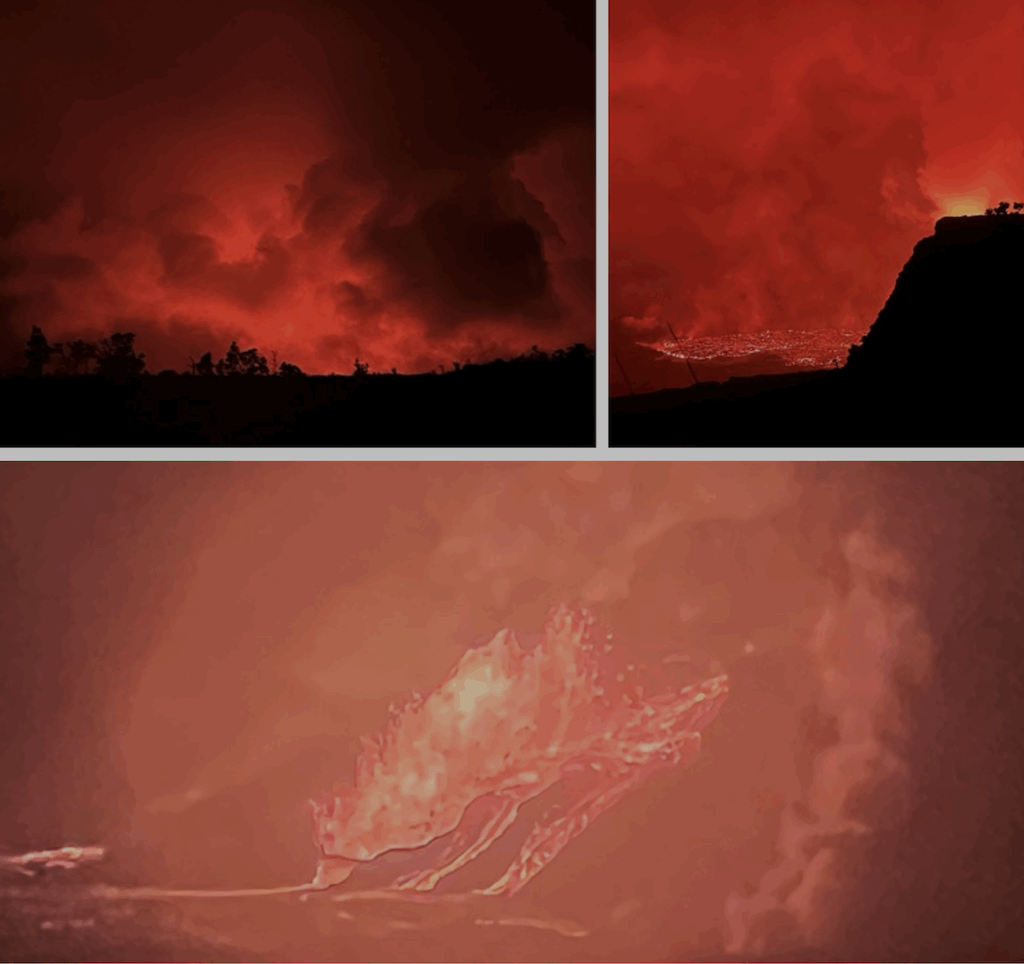
From the caldera below, steam is rising in billows that mirror the cloudy sky.
Day 5 (1/28): Second Eruption and Mauna Ulu
Second episode of the eruption, continued
We awoke, and went straight out to watch the eruption which had intensified over night. We went to Devastation Trailhead and hiked along the closed portion of Old Crater Rim road to where we could get a good view. Two vents were fountaining lava hundreds of feet in the air; it splashed down and flowed into the growing lava lake on the floor of the crater – the lake was dark, but riven with glowing cracks of fire red. At the edges, toes of pahoehoe lapped forward. Overhead a dense twisted column of white smoke rose from the vents and pooled into massive clouds far above. And in the background there is a continuous deep roar, like the rumble of a never-ending train.
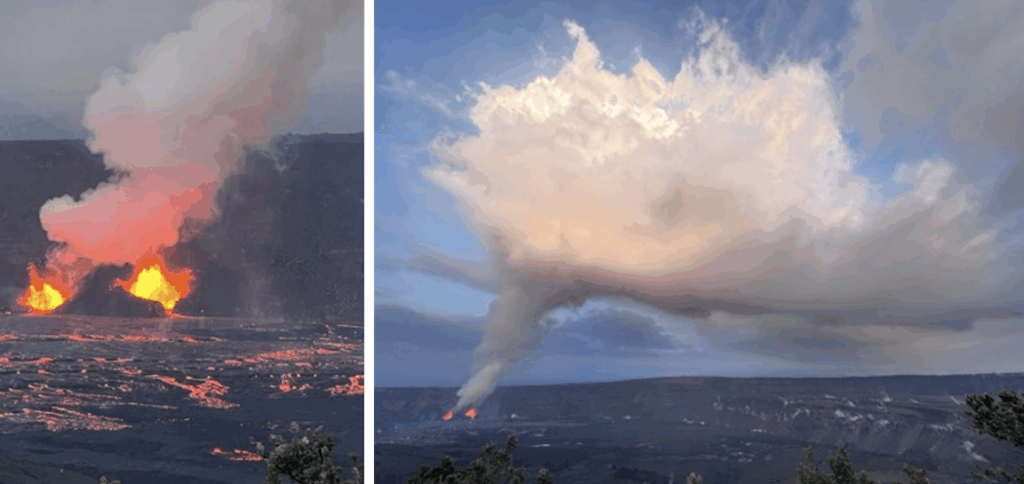
Napau trail to Mauna Ulu Shield
The plan for the morning was to return to Napau Trailhead, where we were yesterday, and hike the trail to the Mauna Ulu Shield. Mauna Ulu is a small shield volcano – known as a satellite or parasitic volcano — in Kilauea’s East Rift zone that was formed by a series of eruptions from 1969 to 1974. It features pahoehoe, a perched lava lake, lava channels, and a crater so deep that we were unable to see the bottom (being wary of getting too close to the edge). On the way there we took a side-trip to Pu’u Hulu Hulu, a fern-filled crater so inaccessible that even wild pigs can’t get there; as a consequence, it is an orchid refuge.
Day 6 (1/29): Kilauea Iki and flux banding
Kilauea Iki
The eruption of the Kilauea Iki pit crater in 1959 created a lava lake approximately 130 meters deep. Over the next three decades, the U.S. Geological Survey (USGS) scientists conducted detailed studies—drilling into the solidifying lava lake to measure temperature profiles, sample cores, and observe the crystallization processes. They discovered that the newly formed basaltic layers continued to circulate internally for a surprising length of time, demonstrating that cooling lava can remain dynamic and chemically evolving well after the surface solidifies. Their research also showed how minerals separate out (fractional crystallization) in basaltic magma chambers, and how gas content, cooling rates, and layering patterns influence the final rock textures.
We hiked into the crater and across the now-solidified lava lake. We saw the vent from which the lava erupted, the main lava channel, and the lake itself. The surface of the lake showed polygonal cracking, with, occassionally, lava ‘squeezing up’ through the cracks. The lava lake also exhibited “overturn,” where the cooling lava on the lake’s surface becomes unstable because of its growing density, and at various points a wave of overturning sweeps the lake and leads to “resurfacing.”
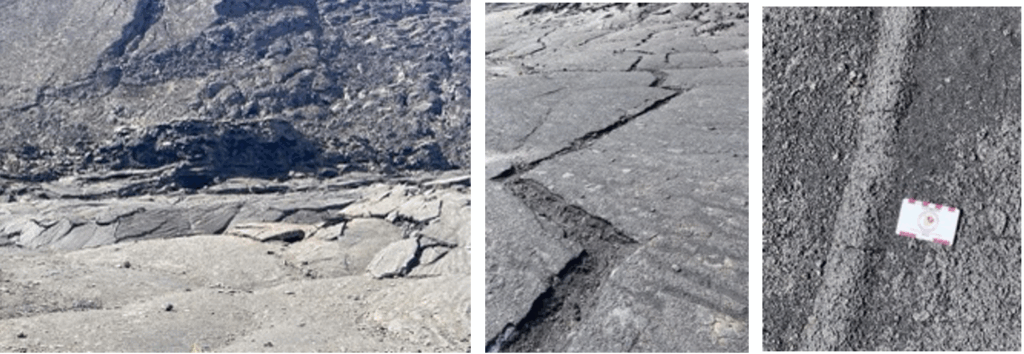
At a larger scale we could see lava islands–portions of collapsed wall that had fallen into the lake (left) – and lava ‘bathtub rings’ (not shown). It was also interesting to see Ohia trees colonizing the lava, growing out of cracks in the basalt.
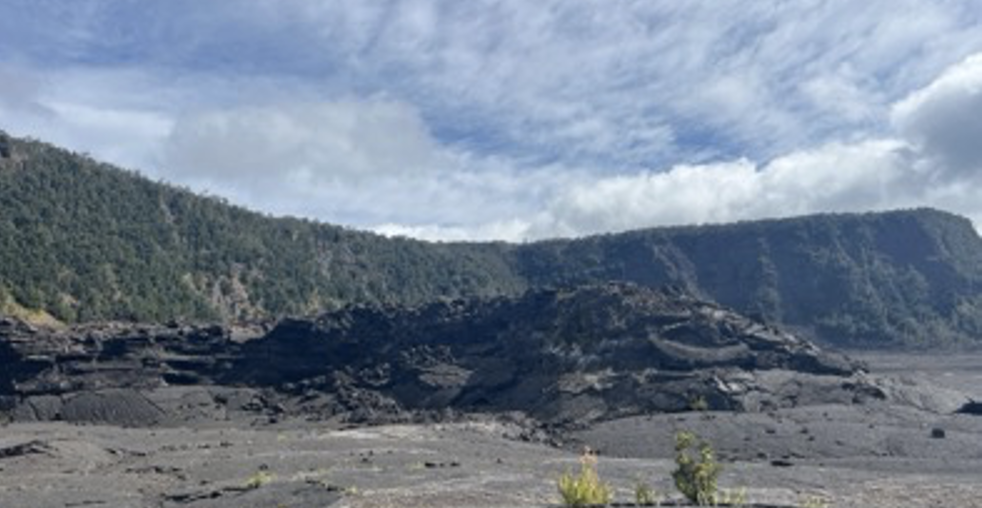
Fault Zone and fluxion banding
In the afternoon we went to examine a fault zone. I didn’t get a lot out of this, but I did notice some unusally patterned basalt and asked about it. I learned that the pattern is called flux banding, and happens as lava or magma is moving over a surface that creates shear; this is exacerbated when the lava includes phenocryst.
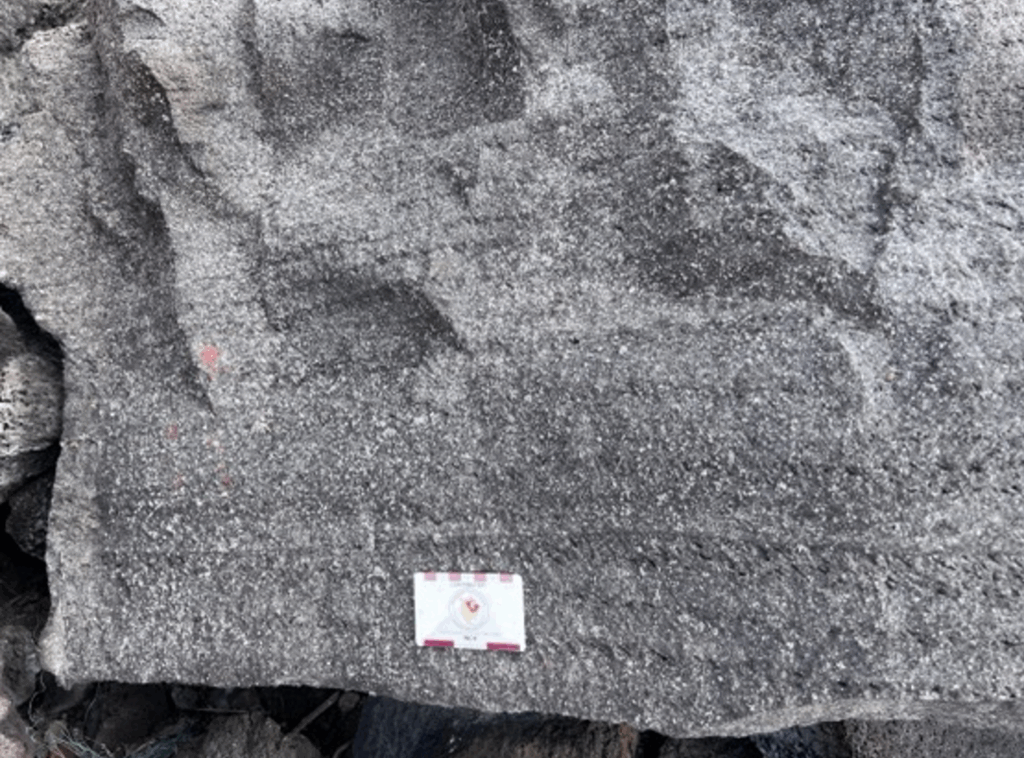
About Fluxion Banding from ChatGPT:
“Fluxion banding is a type of flow-related texture observed in volcanic and intrusive igneous rocks, particularly in lavas and some plutonic settings. It forms due to the differential movement of molten or partially molten material, creating distinct bands of varying mineral composition, crystal size, or vesicularity.
“Fluxion banding results from shear forces within a moving magma body due to:
- Differential Flow in Lava – As lava moves, its viscosity varies due to cooling and crystallization. The outer layers, which cool faster, may develop a plastic or solid crust, while the inner material remains fluid. This difference in viscosity causes layers of magma to stretch and deform, forming elongated bands.
- Crystal Sorting and Alignment – As magma flows, mineral crystals within it may become aligned due to shear stress. This is common in silicic lavas like rhyolite and dacite, where feldspar and quartz can form parallel bands.
- Magma Mixing and Compositional Banding – If two magmas of different compositions mix, they may not completely homogenize, leading to streaks of contrasting compositions that appear as bands.
- Intrusive Settings – In some plutonic rocks, fluxion banding may form as a result of late-stage magmatic flow, where crystals and melts segregate due to convection or deformation.”
The day ended at Hilina Pali overlook — it was a lovely place, and immensely quiet.
Day 7 (1/30): A rainy day for reading
It was raining very heavily in the morning, so we stayed in; I ended up reading quite a bit of the Walker paper o Basaltic Volcanoes. The rain lightened in the afternoon, so we went down to Hilo and saw Akaka falls, and then had dinner at the Ola Brewhouse.
Day 8 (1/31): Lava diversion; black sand; Fissure 8
The day began with periods of torrential rain and wind, plus intense fog; it began to let up around 8:30am. We visited various sites in Northeast Haswaii: We visited the recycling plant that was almost overrun by lava, and looked at how a chain link fence served to (partially) block it. We had a short discussion about lava diversion, and that using a ^ form facing the direction of flow is most effective.
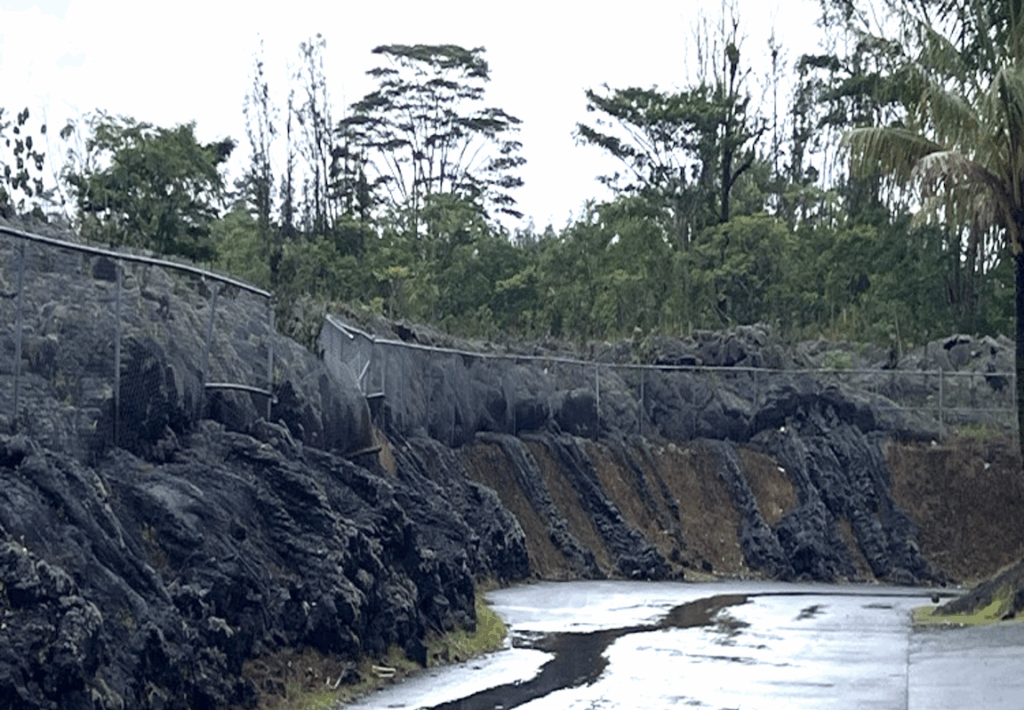
We also visited some black sand beaches and it was interesting to see that the sand includes olvine grains. Also got smoothies at a local place.
Fissure 8
The we went on to Leilani Estates and Fissure 8 – by this time the nasty weather had abated. Our hosts (the Walkers?) enabled us to do the hike to the top of cone around fissure 8, and to view the heavily oxidized interior; on the way back I collected several pieces of reticulite, a stone (or glass) essentially composed of lava foam.
Fissure 8 produced a big a’a’ flow. A’a flows can be divided into basal breccia; top breccia; flank breccia, with a core of unbroken flowing lava. This sort of layered structure—brecciated at the surface and the base with a more solidified interior—is what makes a’a flows stand out in contrast to smoother, rope-like pahoehoe flows.
The eruption of fissure 8 also produced a lot of tephra which forms the elongated ‘cone’ around the fissure; the bottom of the fissure is ‘paved’ with pahoehoe. Fissure 8 was one of 24 fissures that opened during the 2018 eruption.
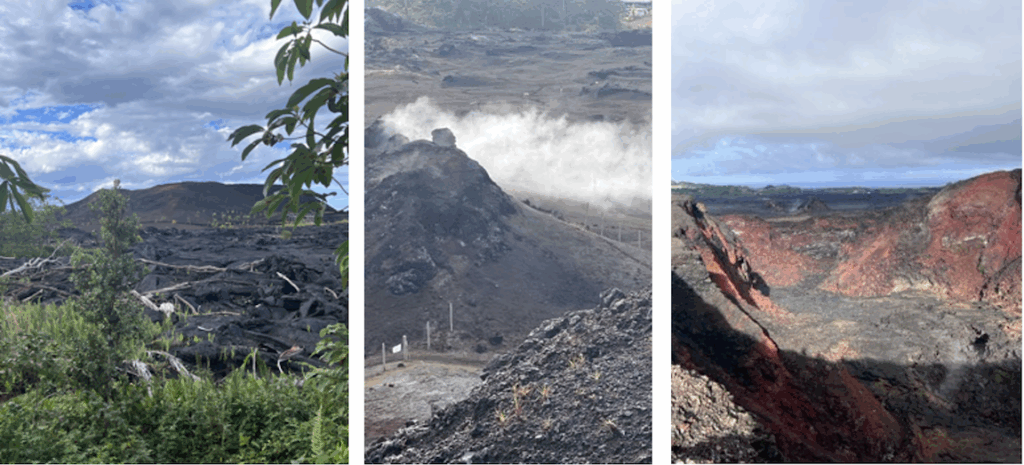
Here is a close-up of the tephra produced in the eruption (picture width: ~3 cm):
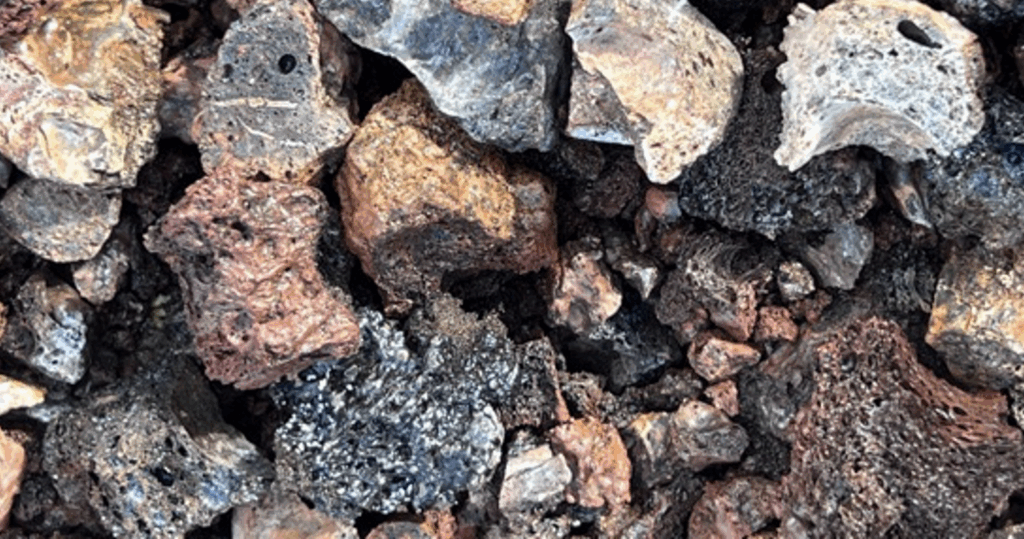
Day 9 (2/1): The Helicopter tour
The helicopter tour was pretty lackluster – a dropping cloud cover prevented us from going very high. We couldn’t even get an areal view of fissure 8, let alone the Kilauea caldera. We did get a good look at Eastern Hawaii, and the various lava flows – there were some pleasing textures and patterns.
I particularly liked the jig-saw-like texture of the forest and the way the canopy shows inter-tree boundaries:
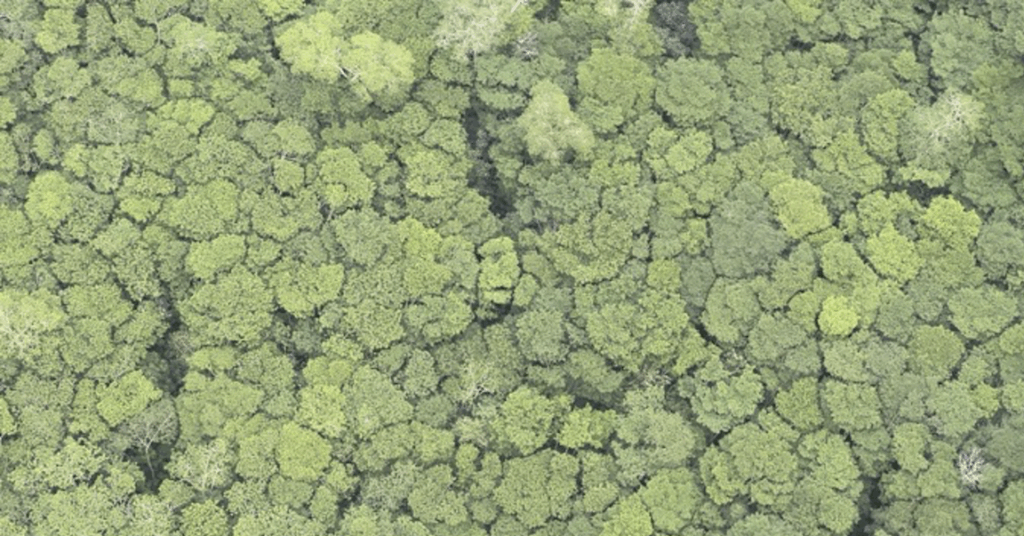
Trees that exhibit this phenomenon are called “crown shy.” These may be Ohia trees, but I’m not certain of that.
The picture of lava channel shows how much it resembles a river; and the second picture shows what is known as “the great crack;” for scale, note the road to its left.
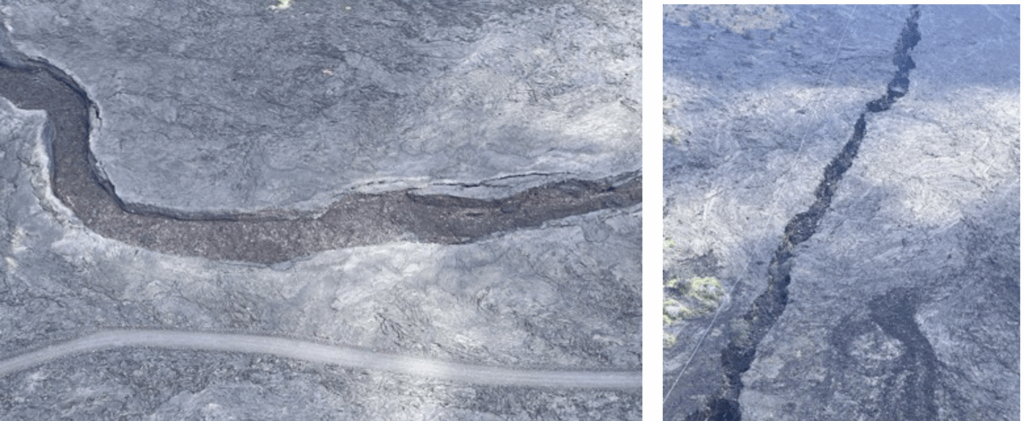
how much they resemble rivers; here, as well, are areal views of a’a and pahoehoe:
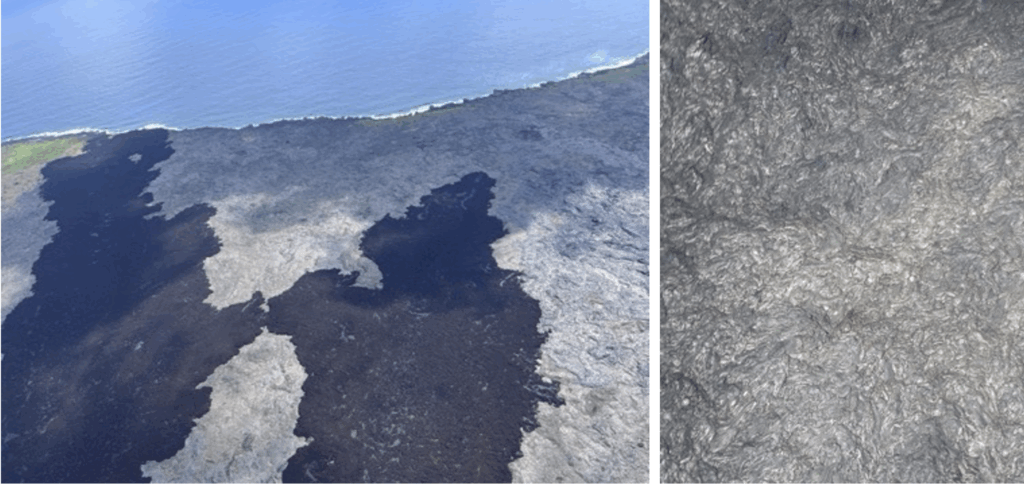
After the tour we had a late lunch at punalu’lu bakery, and then went on to South Point, where we found some patches of green sand, without having to make the trek to the green sand beach which we didn’t have time for.
Day 10 (2/2): Hawai’ite, Phreamagamtism and Peperite
The trip is now shifting its focus away from Kilauea to the other volcanoes: Maua Loa, Mauna Kea and Kohala. The latter two, in particular, are transitioning to their post shield phases, and as a consequence are producing more alkaline lavas, and hence more cinder cones and ash. It is fun to look at the distant profile of Mauna Kea and see how ‘bumpy’ it is – those are cinder cones produced by the more viscous alkaline lava.
Hawaiite
We visited a point on the north coast where there is a flow of Hawaiite into the ocean. I believe it was from Mauna Kea, but am not certain on that. [check] One of my interests on this trip is seeing the different forms of basalt, and the degree to which they can be distinguished visually. Hawai’ite is definitely lighter in color than thoeltic basalts, and its vesicles, from the examples I have seen, are generally larger as would be expected from its higher viscosity.
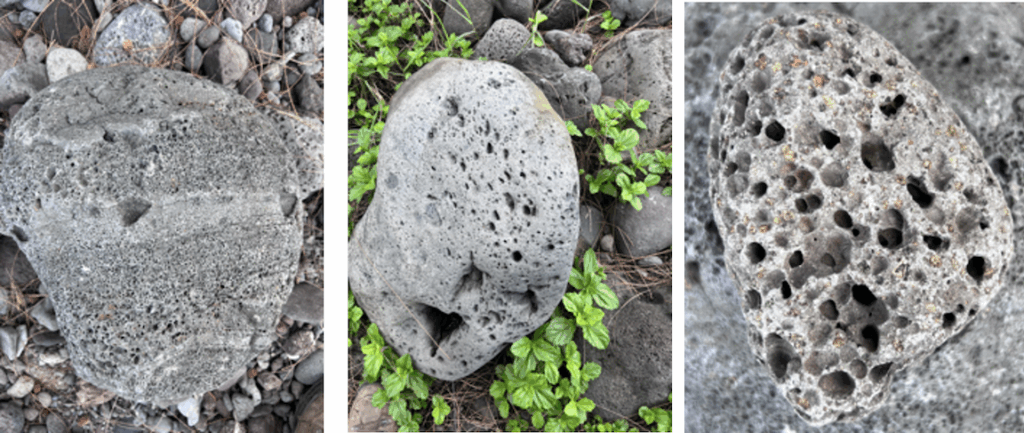
Description: Hawaiite is a relatively evolved type of basalt, meaning the magma that produced it has undergone fractional crystallization, resulting in a higher alkaline content (Na and K), and a somewhat higher silica content. This makes it more viscous, with larger vesicles, and lighter in color than the thoelitic basalts. Hawaiite is commonly characterized by the presence of minerals such as plagioclase feldspar, augite, olivine, and sometimes magnetite.
Phreamagmatic Structures: Tuff cones, Tuff rings, and Maars
The structures produced by the more alkaline lavas are not to be confused with the structures produced by phreamagmatism — eruptions involving the interaction of magma or lava with external water (groundwater, lake water, or seawater). These include tuff cones, tuff rings, maars (diatremes), litoral cones, and rootless cones.
Types of phreamagmatic structures:
- Tuff cone: Steeply-sloped relatively small cone of fine-grained ash and volcanic debris that forms around a central vent; their deposits often reflect repeated pulses of wet ash fall, which can produce well-bedded, fine-grained tuffs. The steep slope is because the wet fine-grained ash tends to cohere. Tuff cones form when magma interacts vigorously with water at or very near the Earth’s surface. Diamond Head on Oahu is an example—its name comes from calcite crystals which were formed later… the abundant calcium coming from its eruption the coral and limestone. A subaqueous tuff cone is known as a Surtseyan cone.
- Tuff ring” Tuff rings have gentle to moderate slopes (often <20 degrees) and are usually wider and have deeper craters relative to tuff cones. Tuff rings typically represent longer-lived phreatomagmatic eruptions that occur below (but still near the surface). They deposit layered, ash-rich tuffs in relatively thin, laterally extensive beds.
- Maars typically have a large, wide, deep crater with a low rim composed of ejected volcanic fragments; the crater floor of a maar often lies well below the original ground surface. Maars form from deeper phreamagmatic interactions in the bedrock that result in violent explosive eruptions that blast out primarily rock fragments, leading to the lower rim.
- Diatremes are a subvertical, volcanic pipes (often carrot- or funnel-shaped) formed by phreatomagmatic explosions/ These explosions fracture the surrounding rock, creating a broad conduit filled with a chaotic mixture of magma fragments, country rock, and juvenile clasts (so-called “diatreme breccia”). Many maars are the surface expression of a diatreme, which is the subsurface structure feeding a maar, continuing downward from the broad crater.
- Littoral cones form when lava enters a body of water or comes into contact with water-saturated ground. Explosive steam generation can hurl molten spatter and fragments upward, forming cones that consist of abundant spatter in addition to ash. They often form when a large front of lava enters the sea, such that not all the lava is explosively converted to ash and tephra.
- Rootless cones form when lava flows over water-saturated ground (like marshes or wetlands). Steam explosions occur within or just beneath the lava flow, ejecting spatter and creating small cone-like features not fed directly by a magma conduit.
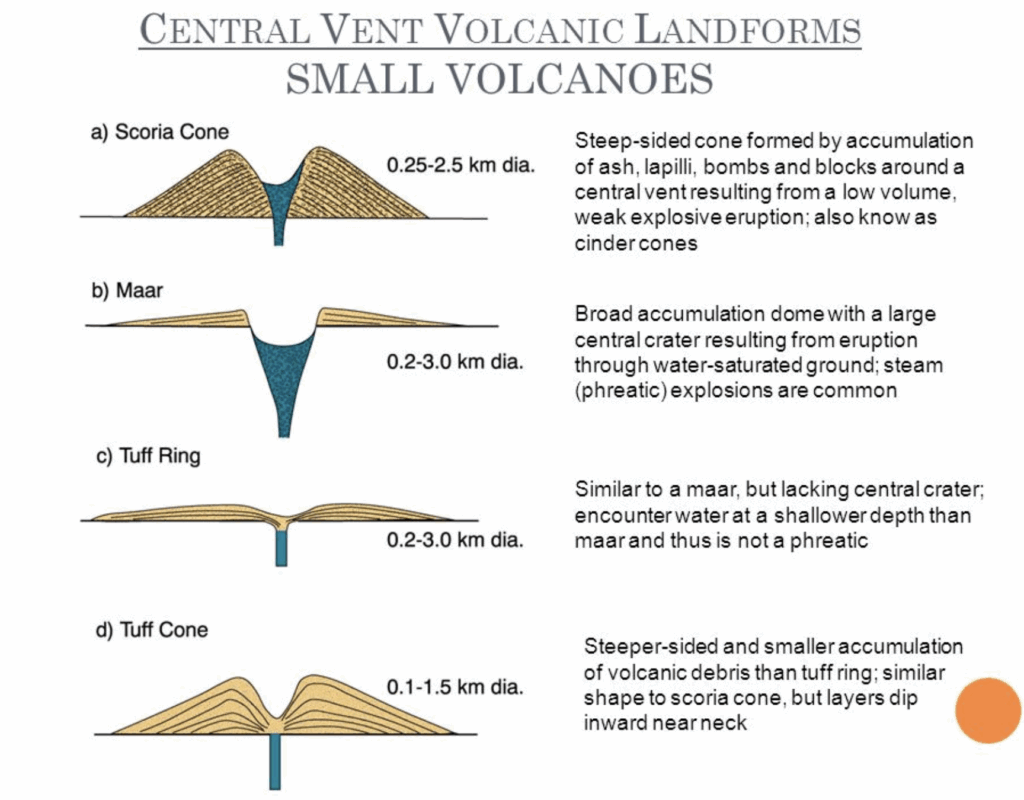
To summarize tuff cones are steeper and smaller, tuff rings are broader with lower rims, and maars form wide depressions often extending below the original ground level—they vary due to the depth at which the phreamagmatic interaction occurs. If there is a lot of lava at the surface, a littoral cone will form rather than a tuff cone (because the liquid lava creates spatter). If there is no connection to a below-ground source of magma, it is a rootless cone.
Peperite
Associated with phreamagmatism is peperite, a magma–sediment interaction deposit formed when magma intrudes or flows into wet, unconsolidated sediments, causing hydrovolcanic fragmentation and mixing of sedimentary and magmatic materials. Pepperite often shows a jigsaw-fit texture or blocky clasts of lava within a matrix of sediment, giving it a unique patchy look. (Note that peperite is primarily a textural/sedimentological term).
One wit described peperite as “a basaltic sneeze into wet sediment.”
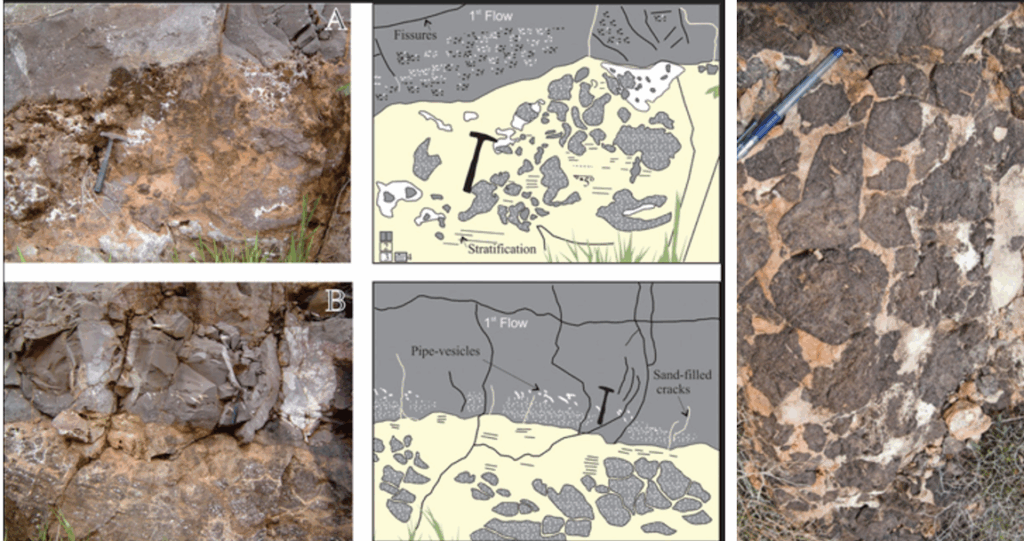
Figure: Peperite layers showing basalt volcanic clasts hosted in eolian sandstone. (a) Peperite texture in very fine grained sandstone with cross-bedding, low-angle stratification with juvenile volcanic clasts of amoeboid shape. (b) Pipe vesicles and sand-filled cracks in the base of the sequences.
Day 11 (2/3): Mauna Loa, Mauna Kea and mantle xenoliths
We drove up a small road to the Mauna Loa summit that was blocked by an a’a flow in 20222.
The first picture shows Mauna Loa in the distance; the bumps on its silhouette are cinder (scoria) cones which indicate that it’s in a later stage of activity and erupting more alkaline (viscous, explosive) material.
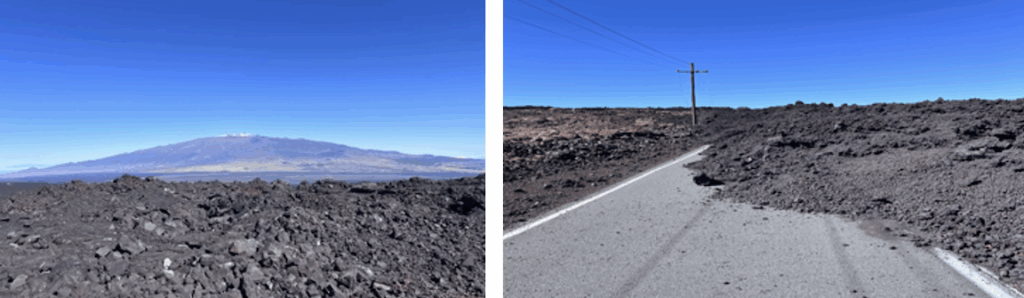
The second picture shows the end of this road to Mauna Loa, as of 2022 a’a flow
Here we see multiple shades of lava; the lighter, more reddish flows are older, having undergone more oxidation:
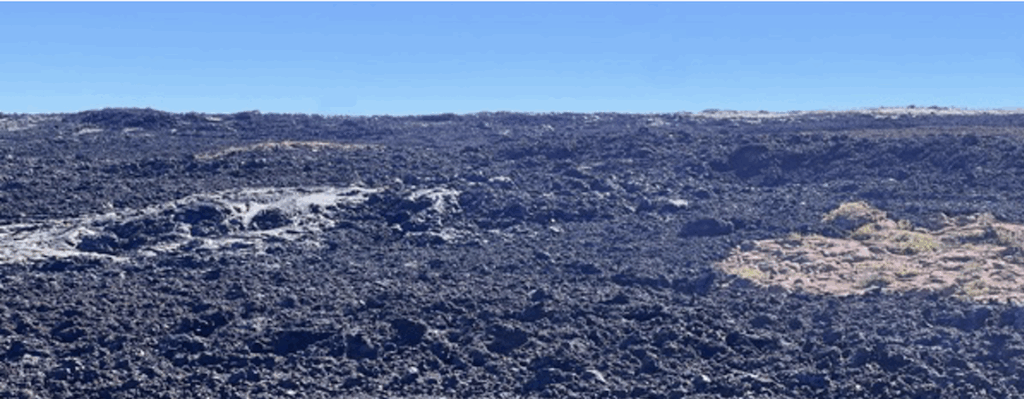
Mauna Kea and mantle xenoliths
We then drove up towards the Mauna Kea summit. We arrived at the Visitor Center at 8000 feet. We only had one vehicle that could go higher due to insurance issues, so I opted to stay behind, having seen the summit on the 2020 trip. I waited with others and spent the time reading, until I learned that cinders from nearby cinder cones had been found to contain mantle xenoliths that contain peridotite, olvine and other mantle minerals. Very cool! So I hiked up to the nearby cinder cone and found a few samples which are depicted below (the xenoliths are crystalline and quite sparkly, though that does not show well in the photos.
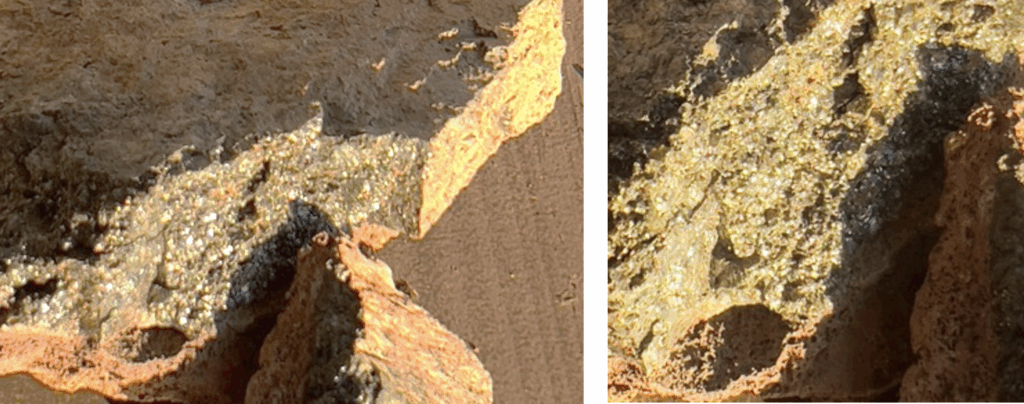
The mantle xenoliths from Mauna Loa are mostly peridotites, with compositions dominated by olivine, orthopyroxene, clinopyroxene, and spinel. Some key types include:
- Dunite (nearly pure olivine). Almost entirely green (deep green to yellow-green) due to its high olivine content; its texture is granular and coarse-grained, with visible rounded or interlocking olivine crystals. May contain small black grains of chromian spinel. The olivine-rich rock tend sto weather to a rusty-brown or yellow-green due to iron oxidation.
- Harzburgite (olivine + orthopyroxene). Greenish with a slight brown tint, and coarse-grained, often with a worn, weathered surface. Contains 20-40% brownish elongated grains of orthopyroxene that may appear shiny or flakey under light. The olivine-rich rock tend sto weather to a rusty-brown or yellow-green due to iron oxidation.
- Lherzolite (olivine + clinopyroxene + orthopyroxene). Mixed colors of green (olivine), brown (orthopyroxene), and black (clinopyroxene); coarse-grained. Because it contains both orthopyroxene and clinopyroxene (in addition to olivine), it has a more diverse color range.
- Wehrlite (olivine + clinopyroxene). Dark green to black, coarse and dense. In addition to olivine, contains 30-60% clinopyroxene; may contain chromian spinel. Often has a more glassy or slightly metallic luster in fresh cuts.
Day 12 (2/4): Kohala: Cinder cones, Muegerite/Benmorite & Ramps
Cinder cone structure
We stopped to look at a cinder (scoria) cone: it is easy to see the cross bedding in the heavily oxidized layers of ash, tephra and bombs.
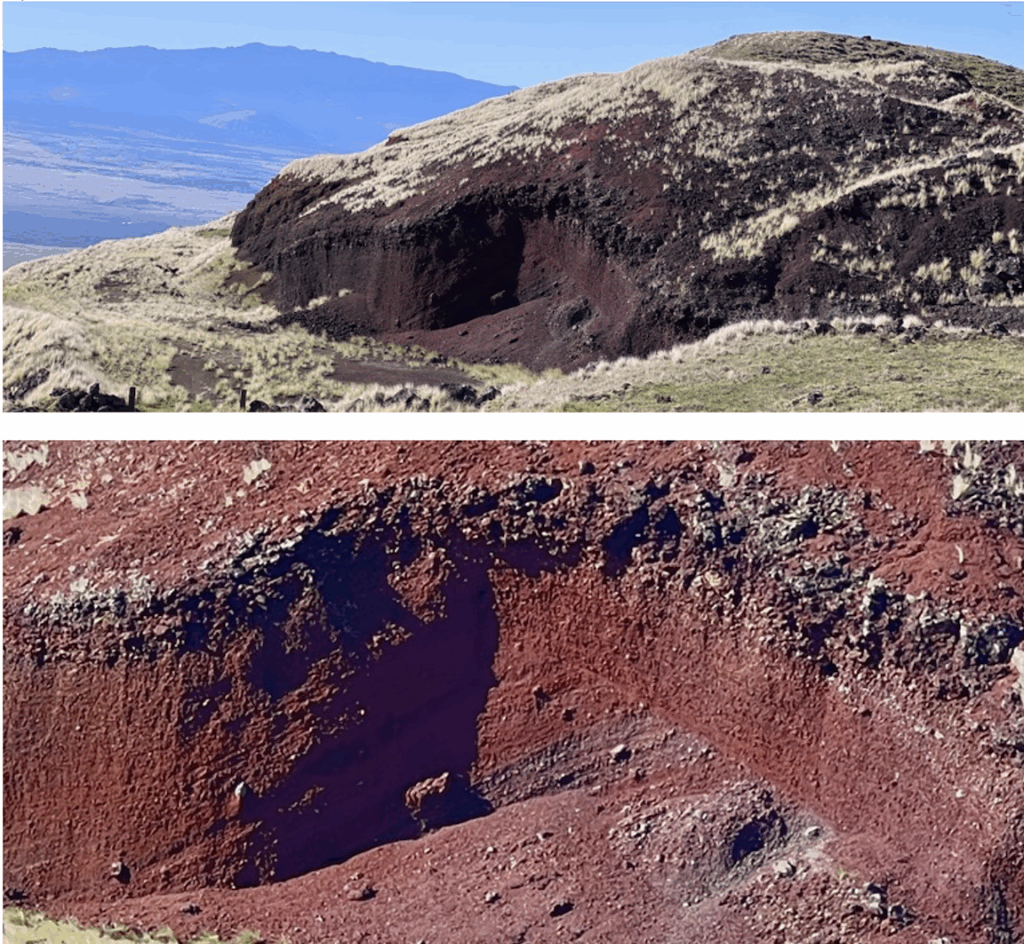
Muegarite and Benmorite
We visited outcrops of two more types of alkaline basalt: muegarite and benmorite. These are even more alkaline than Hawai’ite.
Muegarite has plagioclase and olivine altering to iddingtite (which exhibits cleavage, unlike olivine:
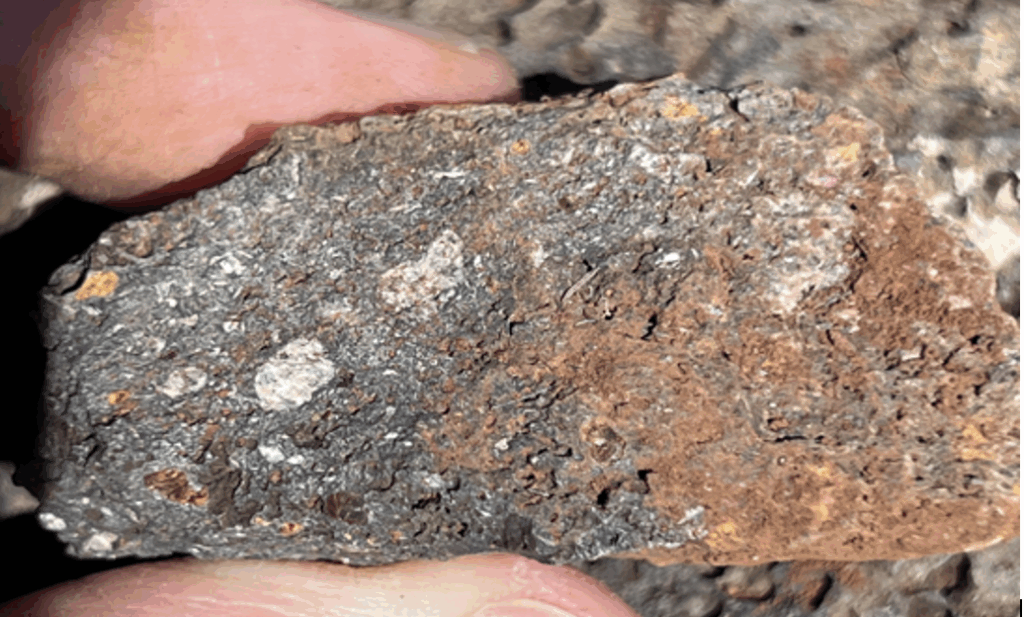
Benmorite – at least the outcrop we found – is the lightest gray of any of the basalts, very fine grained, and more friable than I would have expected. This had no instance of vesicles, so I should not be too hasty in making up a story about the systematically varying appearances of alkaline basalts. I found one sample that was very sparkly – George said something about it possibly having quartz crystals created during the gas phase. [CHECK]
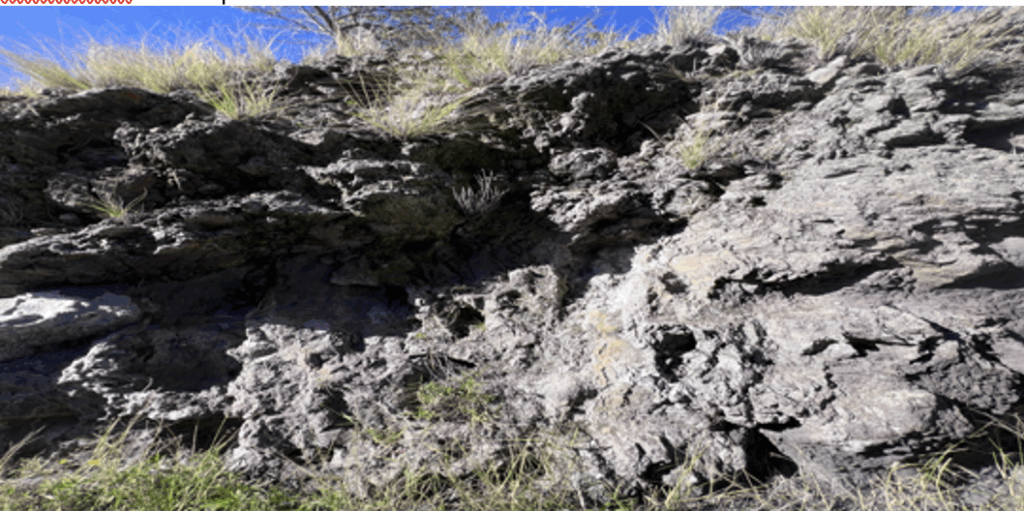
The structure of a’a flows and thrust-fault ramps
The higher viscosity of a’a lava – due to its higher content of crystals, gases, and higher SiO₂ concentration (vis a vis pahoehoe) – restricts its flow, leading to a slower churning movement. The flow solidifies on the surface, but the turbulent flow of the lava underneath causes the surface to fragment into angular blocks and rubble. These pile up on the top of the flow, leading to the characteristic jagged texture; the cooling of the outer crust can also cause convection cells to form below, which can push more lava to the surface, causing it to become very uneven. As the lava cools and the flow advances, these broken blocks are pushed forward and can create lobes and levees, which help channelize the flow.
As an a’a flow moves down slope, the lava at the leading edge is compressed as it is pushed over underlying material. These compressive forces can cause the brittle crust to break, forming thrust faults or ramps. As the lava continues to flow, the solidified lava blocks to “ride over” one another, stacking up in a ramp-like fashion. The resulting thrust fault ramp has a characteristic stepped appearance and is typically seen where the a’a flow has advanced over a pre-existing flow or surface. The blocks of the upper lava may be displaced vertically relative to the underlying units, creating a stair-step appearance along the flow’s edge.
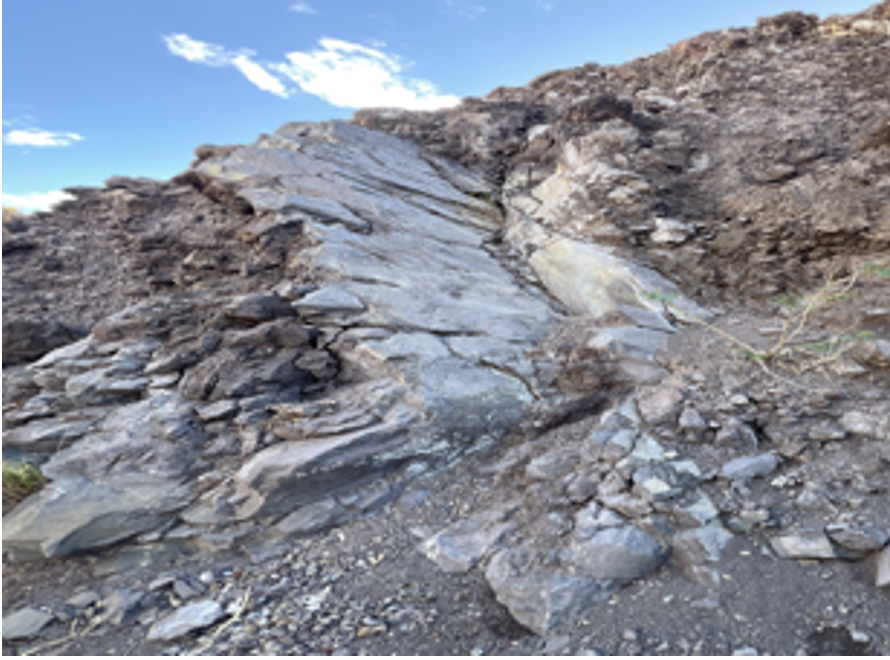
Sheared Basalt
At one stop, I forget which, we saw some ‘foliated’ basalt. This is “sheared basalt.”
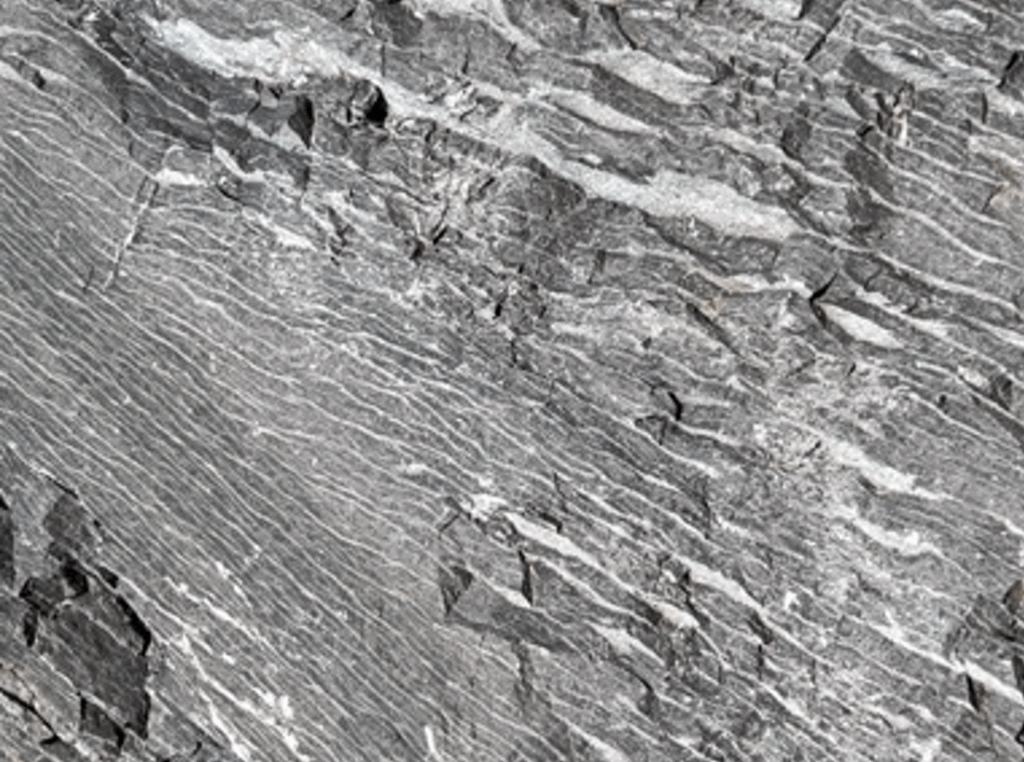
GPT: “When a basaltic body is subjected to tectonic stress—especially in a ductile shear zone—it can become deformed. Under conditions where temperatures and pressures are sufficiently high for ductile behavior (often in the greenschist or amphibolite facies of metamorphism), the basalt will not simply fracture; instead, its minerals may recrystallize or be deformed plastically, forming a foliation. During deformation (and possible metamorphism), new minerals such as chlorite, epidote, and amphibole (hornblende or actinolite) may form. These minerals can grow in an oriented manner, giving a planar fabric. Platy minerals such as chlorite or sheet silicates align perpendicular to the maximum shortening direction, producing the foliation.The very thin layers (on the millimeter scale) often reflect variations in mineralogy that developed during dynamic metamorphism or hydrothermal alteration along shear planes. In some cases, original flow banding in basalt can be overprinted and accentuated by shear-related recrystallization. [CHECK]”
Day 13 (2/5): Last day – Basanite flow with mantle xenoliths
A late start, early end, and a slow day.
The highlight was a stop to see a lava flow of basanite, at Hapuna beach north of Kona: I believe this type of basanite is called Ankarymite, and was from a Mauna Kea flow. [CHECK] I believe it has plagioclase xenoliths. In this flow we also saw more mantle xenoliths (upper right of left photo)
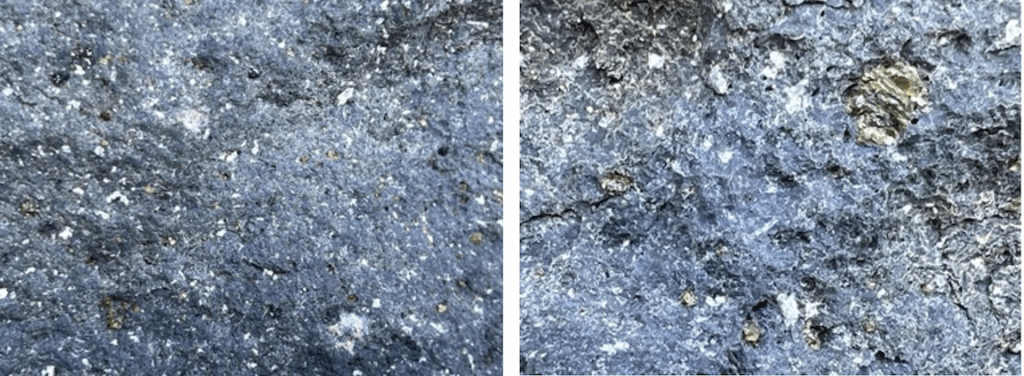
The final stop, which I remember fondly from 2020, was at a Hualali flow – it was, unfortunately, a lot trashier than I remembered.
# # #
Views: 3