Four Billion Years and Counting: Canada’s Geological Heritage. Produced by the Canadian Federation of Earth Sciences, by seven editors and dozens of authors. 2014.
November-December, 2024.
I am reading this with CJS. It is a nice overview of regional geology, and it is nice that all the examples come from Canada, and at least some of the discussion is relevant to Minnesota Geology. The book is notable for its beautifully done pictures and diagrams.
The first part of the book, Foundations, is an introduction to general geological concepts. For CJS and I this will be largely review. Here I will preterit any summary, and simply list some of the points that stood out because they filled in a gap, or provided a different perspective.
FOUNDATIONS
Mostly background on geology for the general reader; but was good as a refresher, and also bringing in North American examples of various phenomena.
C1: On the Rocks
- Polygonal jointing occurs when basalt flows stop moving before they cool.
- Granitic magma forms at 600-900°, sometimes with water contributing to lowering the melting point, where silica minerals tend to melt, but more magic minerals remain solid. The melt tends to move upward, either because it is less dense than surrounding rock or because of tectonic pressures. As it forms a mass, chunks of surrounding “country rock” fall into it in a process called “stoping,” making the melt more silicacious and also persisting in solid chunks that will eventually become xenoliths.
- Ripples (and their large scale cousins, dunes) are straight and symmetric if they are formed by currents moving back and forth, or curved if they are created by a unidirectional flow.
- Mud cracks form as a result of repeated drying and wetting, as occurs in mudflats with seasonal rain, or intertidal areas.
- A seam of coal that is a meter thick was originally 5-10 meters thick and took on the order of 2500 years to accumulate.
- Paleosols, fossil soil surfaces/horizons, are generally rock-like with a characteristic disrupted knobby appearance.
- Metamorphic rocks develop cleavage planes perpendicular to the direction force or pressure is being applied; metaphorphic rocks split along cleavage planes, not their original bedding surfaces.
- Schist has a lot of mica; gneiss has little. Both are coarsely crystalline and so highly-altered that it is difficult to tell what the source rock was. The light and dark banding in gneiss is the result of recrystallization, and has nothing to do with the original bedding plane.
At lower metamorphic grades, platy crystals of chlorite and mica are common. As higher metamorphic grades are reached, minerals such as garnet, staurolite, and sillimanite may form. Such high-grade metamorphic rocks form at depths of 15 to 25 kilometres within the crust. If pressure (usually the result of deep burial) is a major factor during metamorphism, minerals such as kyanite and glaucophane may grow. The blue colour of glaucophane gives rise to the name blueschist, a rock formed under conditions of low temperature and high pressure.
Igneous rocks also show interesting metamorphic changes.When basalt is metamorphosed at low pressures and temperatures, some of its constituent minerals convert to the green minerals chlorite, actinolite, and epidote, producing a type of rock called greenstone or greenschist. At higher metamorphic grades, greenstone becomes amphibolite, a dark green to black rock made up of interlocking amphibole crystals.
C2: The Dance of the Continents
- Reverse and thrust faults are different. They are created by the same array of forces, but reverse faults are steeper (closer to vertical) than thrust faults. …The text doesn’t say where the line is between them…
- Fault breccia. Rock formed of a jumbled mix of sharp rock fragments embedded in lithified rock flour.
- Nice review of minerals: pages 19-21.
- The Greenville orogen underlies Quebec, the midwest US, and stretches into mexico.
All modern oceans contain areas where the lithosphere is thicker than regular oceanic litho-sphere. These areas include island arcs, oceanic plateaus perhaps bearing atolls, and isolated fragments of continental lithosphere such as present-day Madagascar. If subduction continues and these within-ocean features are swept toward the continent, they will ultimately collide with it. Because high-standing islands or plateaus are more buoyant than regular oceanic lithosphere, they will be scraped off the sub-ducting plate and will stick, or accrete, to the overriding plate rather than be subducted. Many mountain belts contain remnants of such former within-ocean features; such remnants are called terranes (a term not to be confused with terrain, which denotes topography). Terranes thus have a variety of origins: they may be continental fragments (microcontinents); former island arcs; or former pieces of thickened oceanic lithosphere such as Hawaii may become if it is accreted to a continent. Many terranes are a mixture of these elements. The convergence and collision of terranes with continental margins commonly leads to the rise of mountains.
Remnants of former deep oceanic lithosphere can be preserved within an ancient mountain system. Such remnants are known as ophiolite suites or ophiolites…
- Rift Shoulder Highlands are mountains produced as a side effect of continental rifting — when the floor of the rift is bulging upwards.
- Basins. Fore arc basins are in front of island arcs; back arc basins are behind them. Fore land basins can form on continents, due to the weight of accretionary material pushing down that part of the continental plate. …Is the Owens Valley in California such a basin?
- The next supercontinent has already been named: Amasia.
…reading break…
C3: It’s About Time
This chapter was mostly review, but a few things stood out — either as needed reminders or new concepts/approaches.
- Fission Track dating is possible with a mineral called Apatite. It contains small amounts of Uranium that disrupt the crystal structure, and whose decay produces scars. The scars ‘heal’ if it is heated much above 100° C, which can help determine, for example, when rock has been exhumed to surface levels and temperatures.
- Thermoclock dating occurs in minerals which have small amounts of Uranium or Thorium. These isotopes decay, releasing Helium, and when the rocks has cooled below a certain temperature (dependent on the particular rock) the He can no longer escape, and thus the trapped He can be measured and the rock’s age determined.
- Cosmogenic dating is possible when cosmic rays strike a rock on the surface and can alter the isotopes in it — for example, Beryllium. This technique can determine how long a particular rock surface (e.g. a glacial erratic) has been facing up.
C4: Fossils and the Brush of Life
Joseph Tyrell’s fishing trip to the Gunflint region — he sampled and later studied a black chert that had xxx Ga microfossils in it.
- Types of fossilization: Permineralization is when pore spaces are permeated by mineral-bearing fluids which leave mineralization in the pore spaces; Replacement is when the material of the bone or shell is replaced by another mineral; Petrification is when both permineralization and replacement occur. In addition, molds or casts preserve the outlines of an organism, and trace fossils preserve a mold or cast of impressions made by organisms’ burrows, tracks, trails or footprints.
…reading break…
Part II: The Evolution of Canada
C5: From Stardust to Continents: Before 2.5 Ga
- 4.567 Ga– – Solar System forms… quite precise for something that would seem to have fuzzy boundaries — did it really coalesce in a million years? …Or maybe that’s the oldest meteorites, and it was when the matter solidified enough to be datable…
- 4.55 Ga — Age of most meteorites , which indicates that solar system did condense quite quickly, but not in a single million years!
- 4.52 Ga — earth and a Mars-size body collided, giving the earth a ring that condensed into the moon; this also gave earth its 17° axial tilt
- Initially the moon was less than a quarter of its current distance from the earth; and the earth was spinning faster, with 6 hour days. (And the sun was hotter as well.) The moon’s outer skin has largely been frozen since its early days.
“The Moon’s outer layers solidified from a global magma ocean roughly within the first 100–200 million years after its formation around 4.5 billion years ago, forming a stable, mostly anorthositic crust by about 4.4–4.3 billion years ago. The lunar mare basalts, which fill the large impact basins on the Moon’s surface, were emplaced significantly later, primarily between about 3.9 and 3.0 billion years ago, with some volcanic activity possibly continuing until as recently as 1.2 billion years ago.” [GPT] - 4.4 Ga — Earth had abundant water. Water carried into magma ocean below by sinking slabs led to a more silicic magma (with less magnesium and iron), and, being less dense, it tended to rise into the crust and form granitic plutons. Over time some would have risen to the surface, forming rafts of felsic rock that eventually got amalgamated into the first cratons.
- 4.3-2.5 Ga — Craton formation
- 3.5 Ga +/- 0.3 Ga — First evidence of life. (1) stromatolites; (2) mudstones with altered C12/C13 ratios indicating biological activity; (3) possible fossils of protists; (4) beginning of banded iron formations…
- >2,6 Ga — komatiitie lavas
- Basalt metamorphoses into greenstone (chlorite, actinolite) and that into hornblende.
- 2-7 – 2.6 Ga — Superior Craton forms — proto-continents welded together with oceanic lithosphere trapped between them.
About 2,750 million years ago, the parts of the Craton underlain by granitic rocks (let’s informally call them protocontinents for simplic-ity) were separated by oceans, the width of which we can only guess. Around 2,720 million years ago, two protocontinents in the north (in terms of modern directions) collided, trapping a mixture of oceanic material between them. Around the same time, other small protocontinents in the south amalgamated and were welded onto the northern block, sweeping up more tracts of oceanic lithosphere in the process. This amalgamation process, during which the oceanic material became compressed into the greenstone belts, continued until about 2,680 million years ago, by which time all the pieces of the Superior puzzle had come together. - 3.8 – 2.5 Ga — BIF (Banded Iron Formations). Differing theories for BIFs. One is that cyanobacteria produced O2 which led to oxidation of iron in ocean; (2) another is that early single-celled organisms used iron “to provide electrons for photosynthesis” producing oxidized iron.
…reading break…
C6: Laying the Foundations: 2.5 Ga to 750 Ma
- Stanley Tyler discovers outcrop of Gunflint chert that is black and waxy-looking: This 1.9 Ga chert contains micro-fossils: thread-like filaments and hollow spheres on the order of a few microns across.
- 2.5 – ~2 Ga — Breakup of Archean protocontinents (Slave and Superior cratons are remnants of these. This was accompanied by the formation of gigantic swarms of radiating dikes, possibly due to mantle plumes (e.g. Matechewan dike swarm in southern part of the Superior craton.
- 2.5 – 2.4 Ga — The Great Oxidation event forms (among other things) beds of red sandstone. Note that the oxygen content of the atmosphere was still far less than it is today — a few percent (vs. 21%)
- 2.45 – 2.2 Ga — Huronian tils provide evidence of first glaciation (in three waves)
- 1.8 Ga – Nuna, first supercontinent forms.
- 1.85-1.2 Ga — island arcs accrete on the southeast edge of Nuna. Also, in the interior, mountain ranges are weathering and sediment is being deposited in interior basins (e,g,, the Thelon and Athasbascan basins).
- 1.09 – 1.0 Ga — Greenville orogeny (which involved collision of Amazonia (part of South America) with Laurentia) focused along what is now the southeastern flank of the Canadian shield. This was part of the assembly of Rodinia, and also possibly the genesis and termination of the mid-continent rift.
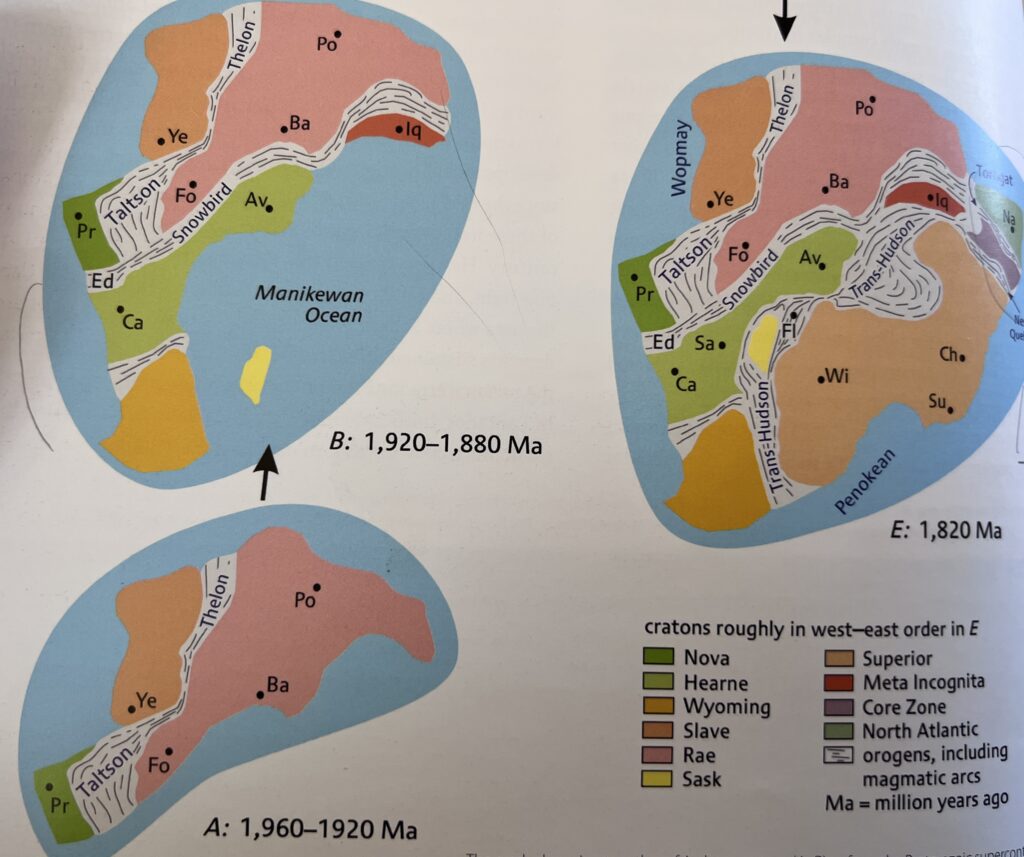
- 1.0 – 0.750 Ga – Rodinia.
- Passive margin sequences, from bottom to top (e.g. Huronian sequence in southern Quebec and Ontario exposed along trans-canada highway between Sudbury and Saute Ste. Marie):
- rift-related coarse-grained sedimentary rocks possibly interlayered with or intruded by mafic volcanics. This stage of rift-filling often includes deposits of dense placer materials (e.g. uranite and pyrite) deposited where flowing streams slow down.
- as the rift widens and sea floor spreading begins to form an ocean, there are finer grained and better sorted sandstones and mudstones.
- as the ocean matures there are carbonate and chert layers (with stromatolites) from the shallow ocean floors
…reading break…
C7: Southern Sojourn: Canada 750 to 444 Ma
- Discovery of Precambrian multi-cellular fossils in Newfoundland: aspidella and charniodiscus.
- Cryogenian (850-630)=>Edicarian (630-542) =>Cambrian 542-488) =>Ordovician (488-444)
The Cryogenian
- Rodinia => 780-750 Ma breakup…
- Laurentia a separate continent by … experts disagree: 750-520 Ma. Anyway, over time, Laurentia, what was to become North America, was rotated 90° so that what is the east coast was oriented towards the paleosouth.
- Windemere seaway rocks. A stack of sedimentary rocks that accumulate in a trough from California to Alaska — it is now found along the easter Cordillera. The stack includes deep water clastics, carbonates, and tillites with dripstones that were deposited in a rift basin (that perhaps evolved into a passive margin) — it can be seen in road cuts between Jasper, Alberta and Tete Jaune Cache BC, and also near Lake Louise.
- Snowball earth? Tillites and dropstones occur at 740-670 Ma and 635-600 Ma. Paleomagentic data indicates that some of these sediments formed within 10° of latitude of the paleoequator. This is evidence for global glaciation, though many geologists are skeptical and favor a scenario where there re tracts of open ocean in which organisms would have been able to survive.
- (If the earth was entirely glaciated, CO2 from volcanism would build up (because it would not be absorbed by the ocean or by surface weathering) and eventually a greenhouse effect would kick in).
The Edicarian
- Metasoans appear ~ 580 Ma. Life prior to 580Ma (late Edicarian) was dominated by single-celled organisms; metazoans (multicellular animals with soft tissues in the form of disc-shaped, segmented or frond-like organisms) appeared as evinced by centimeter to meter scale fossils preserved in layers of sandstone or volcanic ash.
“While glaciers coated the world, the only organisms that fed on light beams were cyanobacteria. They thrived better than others in the oxygen-poor ocean, a relative nutrient desert. Only the freezing outflows of meltwater rivers carried enough oxygen into the seas for aerobic life to survive. As the snowball began to thaw, the melting ice wore away the continental surface, and drove millions of tons of phosphate into the ocean, creating a chance for an algal takeover. Suddenly, the advantage that cyanobacteria held – being able to absorb nutrients quickly because of their small size – was no longer relevant. Larger organisms were no longer outcompeted. and cyanobacteria were numerous enough to be preyed upon by larger microbes. Being big is an advantage for a predator, even one [that preys] on the microscopic cell, and it is at this time that multicellular algae became common .
– Thomas Halliday, 2022, (Otherlands, 273)
and also
“ In places the ground is coated with a firm, wrinkled layer, hardly distinguishable from the rest of the seabed except in texture, the folds of a rough rhinoceros-hide texture contrasting with the fresh-poured caster-sugar smoothness of ground quartz sand. The rough texture is the microbial mat, coating the interface between earth and water, an ecological structure that has already existed for billions of years. The two simplest domains of life, bacteria and archaea, have fed and reproduced at Ediacara for thousands of years, building layer upon layer as they stabilize the seabed, forming a coherent uppermost layer, like a skin on cold custard. Where the microbes are cyanobacteria, the mats often form distinct clumps called stromatolites that resemble slimy, slowly growing boulders, climbing their way to the light. Elsewhere, as in this delta, the mats are spread flat, a sheet of wrinkled life on the floor of the ocean. Only the top few layers are ever alive at any one time, but the combined generations of millions of microscopic cells nonetheless mean that the mats can reach thicknesses measured in inches.
From these patches of microbial mat, strange, feathery shapes rise up to 30 centimetres into the water. Ridged, rugby-ball-shaped creatures, only a centimetre in diameter, drift between them. Above these hovers an eerie cone, a centimeter-scale flying saucer, spinning as it drifts, before settling once again on the seabed. Close-up, it becomes apparent that this shadowy form is made of eight ridges, spiralling clockwise from the tip of the cone to its base, a coiling helter-skelter; floating hypnotically. Unable to move with great speed in the water, and hardly a natural swimmer, it nonetheless occasionally leaves behind its home on the microbial mat. It is found in the peace and quiet of the calm water below the storm base,and, when swimming, hangs in the water over stranger creatures still. Already, multicellular life is complex, and this is in fact one of the earliest creatures we can with certainty call an animal. Eoandromeda – so called because when flattened in fossilization, its eight arms resemble the spiral galaxy Andromeda – is a lantern in the Ediacaran murk, one of the few forms of life here that is remotely recognizable.”
– Thomas Halliday, 2022, (Otherlands, 275-6)
The Cambrian
- The Cambrian Explosion, 542 Ma. Most sessile organisms became extinct by the end of the Edicarian, possibly due to the evolution of mobile organisms with a head end that allowed them to move forward. The first organisms with skeletons appeared around 550 Ma. The beginning of the Cambrian is marked by the appearance of trace fossils of worm burrows, and that was followed by the first “shelly” fossils. After that came brachiopods and archaeocyanthans, and then trilobites.
- Oxygen explosion? One possible driver for the Cambrian explosion is that Oxygen increased to 3-10% (15-50% of present-day values). This is consistent with what analysis of contemporaneous sedimentary rocks show. Of course, it is also possible that the evolution of new morphological features — heads, eyes, mouths, teeth, segments, legs and antennae had something to do with it. …These features could have led to an evolutionary arms race as predators and prey engaged in a red queen’s race.
- Protichnites. In 1983 trace fossils of an arthropod walking on land were discovered in a quarry nears Kingston, Ontario, in 500 Ma sandstone formed from aeolian dunes. This is tens of millions of years before any other animals are known to have walked on land, so perhaps this was just a temporary outing!
- paleo-South: Rifting / opening of Iapetus (circa 600 Ma), and microcontinent suturing. Around 600 Ma crustal rifting began to separate Laurentia from Amazonia, forming rifts that can still be seen in the valleys of the St Lawrence and Ottowa rivers. As time went on (moving into the Ordovician) microcontinents — Gander, Avalonia and Magma accreted to the paleo-southern shore of Laurentia (now east coast of North America).
- paleo-East (north): Spreading opens Ural Ocean (circa 600 Ma). From 600-480 Ma this was a passive margin that accumulated carbonates which are still preserved as flat strata overlying artic islands. This margin area may have been quite shallow, as there are extensive deposits of evaporites. Between 480-450 Ma a subduction zone and magmatic arc developed in the Ural ocean, leading to the creation and eventual accretion of amicrocontinent called Pearya to the paleo-east side of Laurentia later on…
- paleowestern (southern) margin. The core of Laurentia has been stable since the Grenville Orogeny — it is called the North American Continental Platform.
Ordovician
- Brachiopods proliferated and nauteloids rose to prominence. Trilobites continued to thrive, as did echinoderms, especially crinoids.
…reading break…
Box 6: The Burgess Shale
- The Burgess Shale (named for a nearby mountain and pass) is near the town of Field in the Southern Canadian Rockies and is renowned for its preservation of a menagerie of 505 Ma fossils of soft-bodies animals. it is now a UNESCO World Heritage Site.
- Fossils in an area called “the trilobite beds” were discovered near Mt Stephen during the construction of the railroad, and Canadian paleontologists identified and characterized a number of the fossils. But it was not until Charles Walcott, an American paleontologist and secretary of the Smithsonian Institution, did further exploration in the area, that the Burgess Shale itself was discovered. Between 1910 and 1924 Walcott and his family collected around 65,000 specimens (!!!!!) from the shale.
- The Royal Ontario Museum’s Burgess Shale collection contains more than 150,000 fossils.
- The Burgess Shale has yielded fossils, often exquisitely preserved, even to the extent of preserving gut contents, of more than 200 organisms, most of which lack shells. The fossils yield great insight about the morphology of Cambrian organisms: some appear familiar to us, others demonstrate mixtures of body parts that strike us as odd and outlandish. For example, Opabina has five eyes, an extended proboscis, a segmented body, and no legs.
- The Shale also offers interesting case study in how fossils have been reinterpreted over time. For example, Anomalocarius Canadences (“Canada’s odd shrimp”) turns out to have been the claw of a larger organism (but which still retains the original name). Similarly, many of the fossils which were once considered to be representatives of extinct phyla are now thought to be early branches of existing phyla.
- Stephen Jay Gould’s book, Wonderful Life, is about the Burgess Shale.
- The Shale appears to be the result of periodic mud slides in the ocean that buried and instantly killed (because no evidence of escape attempts) the organisms, and preserved them in an anoxic environment.
Box 7: The Green Revolution
- Chloroplasts were originally prokaryotic cyanobacteria that were engulfed and incorporated into early plant cells. Note that some cyanobacteria still survive independently.
- Several lineages of algae developed multi-cellular forms independently. They also trapped or incorporated different pigments — red and brown chlorophylls are predominantly found in sea weeds; green is as well, though it is also what gave rise to land plants.
- Freshwater forms of green algae invaded land on many occasions, but only one — embryophytes, was really successful on land. The key was adaptations that allowed plants to live in open air, and deal with dessication. In water, plants can easily obtain food and water by absorption directly into their cells, expelling wastes which diffuse away, and enjoy protection from UV light and neutral buoyancy for support. None of these advantages obtain on land.
- Embryophytes include plants from the simple lungwort to the deciduous trees.
- The first land plants were likely bryophytes such as mosses — they don’t have a vascular system or roots, and thus require moist environments, but they do use spores as a reproductive mechanism. These appeared in the Ordovician, about 470 Ma.
- In the Silurian, at about 425 Ma, tracheophytes — plants with a superior vascular system — appeared. This includes most of the plants we’re familiar with, from ferns to trees. The vascular system both provided means for transporting water and nutrients within the plant, and also mechanical support so that they could compete for light and withstand environmental forces.
- Leaves, wood and seeds appeared in the Devonian (xxx Ma)
- Leaves may have been a response to declining CO2 levels during the Devonian and enabled the plant to take in sufficient CO2.
- Wood enabled plants to grow taller, and by the late Devonian forest canopies had replaced knee-high thickets.
- Seeds, by providing a store of water and nutrients, enabled plants to reproduce in drier environments (e.g. where there were seasonal rains and dry periods.)
- Around the Carboniferous-Permian boundary (300 Ma), the global environment became much drier, and conifers and cycads became the predominant phyla.
- Later (140 Ma) flowering plants came on the scene, and are now one of the most successful types.
- Grass, a type of flowering plant but different in that it is predominantly wind-pollinated, appeared only 25 Ma during the Oligocene. It was adapted to the dry environments of continental interiors, possibly because of a different photosynthesis pathway. [check]
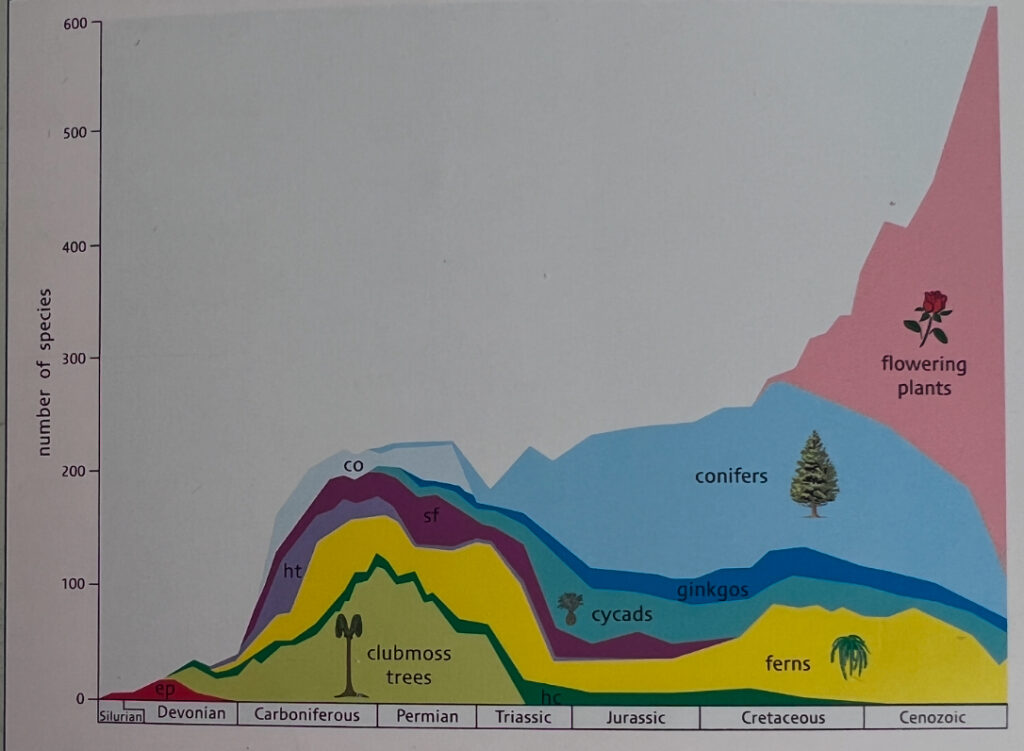
C8: Crossing the Equator
- This chapter:
Silurian(444-416)=>Devonian(416-359)=>Carboniferous(359-299)=>Permian(299-251)- Laurentia fused with Gandara and Baltica to form Euramerica, which then began to rotate [???] counterclockwise until its present eastern margin faced paleosoutheast. The most important point is that what was to become north america was actively rotating and drifting north. By 315 Ma the paleoequaor was crossing through the SW US and eastern Canada.
- 310 Ma: Euroamerica and Protogondwana had come together, forming Pangea, though Siberia was a bit late to the game.
- Collisions
- 1. 480 Ma: Dashwood microcontinent accretes to south short of Laurentia in the Taconic orogeny, the first in the series of orogenies that produced the Appalacheans.
- 2. 440-422 Ma: Gandaria collides with southern Laurentia in the Salinic orogeny. Then in 420-390 Ma Avalonia collides with the Gandarian margin of Laurentia in the Arcadian orogeny.
- 3. 390 Ma. Meguma is pulled sideways against Avalonian margin in the neoarcadian orogency
- xxxx
…reading break…
C9: Pangea Breaks Up and Mountains Rise
- This chapter:
xxx(444-416)=>Devonian(416-359)=>Carboniferous(359-299)=>Permian(299-251)- Overall: Subduction on the ‘west’ coast, rifting on the ‘east,’ a basin with rifting & complex tectonics on the ‘north.’
-
- xxxx
C10: Final Approach: Canada 65 Ma to Today
There is a lot going on here, and to be honest I haven’t tried to follow precisely what is happening when and where. But I learned a lot from this chapter, and will try to capture the various aha’s, and other high points. One nice thing about reaching this point in the book, is that North America, and its global context, is looking pretty familiar — it is interesting to be able to look at landforms and terraines that are recognizable today, and understand the processes which produced them.
Interesting bits:
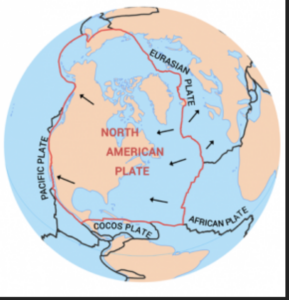
- NA/Eurasian plate boundary. The western boundary between the North American plate and the Eurasian plate is in Siberia! I’d never really though about where it was, but I guess I’d assumed it ran along the west Pacific rim. But the northern Pacific is complicated, in terms of plate tectonics, and just now, looking at maps of the plate boundaries, I notice that maps often don’t offer a continuous view of North American and Siberia/Russia/Asia. But here’s one that does:
Some other maps also show the northern parts of Japan as being on the North American plate, although this one does not. - Land routes to NA. There were, at various times, three land routes to North America. The well-known Beringia route; the isthmus between North and South America, which before it became completely consolidated offered an island-hopping route for some species, and a bridge between NA, Greenland and Scandinavia that existed until about 46 Ma.
- Changes in Oceanic Currents. During the 65 million year period, continental movements had significant effects on the climate. In particular, 30 Ma, the opening of the Drake passage separated Antarctica from South America, enabling the antarctic circumpolar current which caused cooling; this was possibly aided by the north-ward drift of Australia, which constricted the flow of warm water between the Indian and Pacific oceans. More recently, around 0.8 Ma, the joining of North and South America interrupted the circulation of equatorial waters.
- West Coast tectonics. One thing I’ve learned over the last year is that the west coast of NA is composed of a bunch of sutured terranes and island arcs, and that episodes of subducted oceanic crust create magmatic arcs farther inland. In addition, areas in which there is convergence and subduction/suturing alternates with areas where there are transform boundaries (perhaps due to the subduction of spreading rift zones?). This story is repeated up the NA coast to Alaska. It was also interesting to see that there is at least one other hotspot under the NA place in addition to Yellowstone. And finally, as subduction occurs, there are frequent episodes of the formation of accretionary wedges that sandwiched in between terranes.
- Faults, Crustal Thinning and Causality. A passage in this chapter commented that the occurence of normal faults led to crustal thinning — I’d always envisioned it the other way around, that the stretching of the crust led to normal faulting to releave the stress. But the passage made it clear that, at least in this case, tectonic movements created normal faults, and the normal faults in turn led to the stretching of the crust. I wonder if this is always the direction of causality, or if sometimes thinning crust can cause normal faulting.
- Rocky Mountains – ?High? The Rocky Mountains formed 60 Ma, and in the general scheme of things should have mostly eroded by now. But there is apparently some — as yet unknown factor — that leads to their uplift, and thus their continued high altitude.
- River System Reconfiguration. Over this time period, the interior of the Pacific and Canadian Northwest was uplifted, reconfiguring the river systems from flowing to the north to flowing to the south: traces of this can be seen, in some cases, by the acute angles of tributaries flowing into larger rivers.
- Arctic Islands as Submerged Fluvial Terrain. The many small islands that can be seen in the northern Canadian arctic are due to submerged river systems.
…reading break…
C11: The Ice Age
From 2.x6 Ma until 14 Ka North America experienced an ice age during which the ice caps and glaciers expanded and contracted many times – at least 22 – averaging about 41,000 years between interglacial periods.
Glacial Geomorphology. I won’t go deeply into this, as I’m mostly familiar with it, but will highlight what I did learn
- Fossilization. One thing this chapter made me wonder is how quickly fossils can form, since it reports the finding of numerous fossils that indicate ice age conditions. It appears that fossilization can happen very rapidly — on the order of months to years — if conditions are just right, and the organism is small or otherwise suited to it. In the lab, simulacra of fossils have been created in a few hours.
- Drumlins vs. Roche mountonnes. I wondered about the difference in shape between drumlins and roche mountonnes: the first is steepest at its upstream/hill side (stoss), and the latter is steepest at its tail (lee). The difference has to do with the process of formation
- Drumlins are deposits of glacial sediments formed beneath the glacier. The pressure and flow dynamics create a steeper slope on the upstream side (like a ship’s bow) facing the oncoming ice and a gentler slope on the downstream side.
- Roche mountonnes are sculpted out of bedrock. High pressure on the upstream (stoss) face results in abrasion, creating a smooth surface. On the downstream side (lee), lower pressure allows cavities to form between the ice and bedrock, promoting quarrying or plucking of rock fragments along lines of weakness such as joints.
- Ice caps/fields vs. Valley glaciers. Each produce (somewhat) different landforms. A valley glacier will excavate a valley into the classic U-shape, with a cirque at its head; if there are glaciers in adjacent valleys, the adjacent cirques will leave narrow ridges called arêtes, and the glaciers, if they merge lower down, will create medial moraines. When the lobes of ice fields merge, they create interlobate moraines, broad areas of hummocky rocky ground consisting of both til and sand.
- Permafrost landscapes. “A distinguishing feature of continuous permafrost is polygonal patterned ground, the surface expression of intersecting ice wedges. Ice wedges form as the ground contracts and cracks from the cold, forming a void that penetrates the frozen substrate beneath. Snow falls into the crack, melts the following spring, and then refreezes, gradually wedging the ground apart over hundreds to thousands of years. Small ice-cored hills known as pingos also occur in the continuous permafrost zone. They result from the upward bulging of near-surface frozen ground above the ice core. Another feature involves exposed bedrock that becomes shattered into blocks, resulting in a loose, rocky, more or less level ground surface known as a felsenmeer (“sea of rocks” in German).”
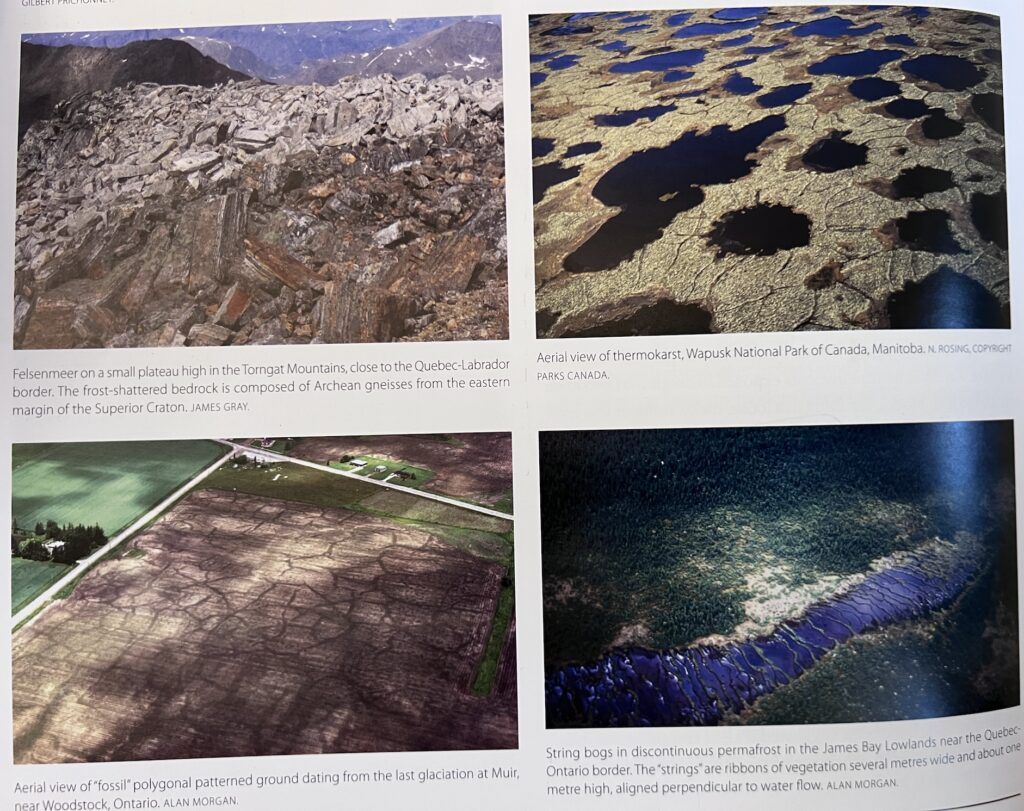
- Milankovich cycles: 100 Ka (eccentricity–closeness to sun) [+] 40Ka (obliquity–wobbling tilt of earth) + 20Ka (precession-)… taken together do a pretty good job of explaining ice age timing, although other factors are in play as well.
…reading break…
- C11, part 2, TBD
C12: TBD
C13: TBD
Cxxx: TBD
Views: 14