* The Master Builder: How the New Science of the Cell is Rewriting the Story of Life, Alfonso Martinez Arias, 2023
March 2023…
I am reading this book with RB, a chapter or two at a time. My first impression is that it is going to be a great read. It has interesting and new-to-me science straight from a scientist who has spent his career studying this area, and it is well written too.
The premise of the book is that DNA gets too much credit for its role in shaping organisms, and that it is also important to pay attention to the ways in which cells carry out the ‘instructions’ of DNA. Unlike DNA, cells can respond to their environment, sense ambient conditions at the cellular level, and respond to distance and orientation. Quite a number of things that we would presume would be determined by genetics — everything from finger prints and retinal patterns to birth defects, propensity to diseases, and the location of organs in the human body — are determined by cells rather than DNA.
Introduction
- Story of Karen Keegan, a woman whose DNA test showed that two of her three children were not hers, even though she and others knew they were. It turns out, that she is a genetic chimera with two different genomes resulting from two of her mother’s eggs fusing during pregnancy.
- It is estimated that 15% of humans are chimeras, although sometimes this is only manifested in blood cells.
- Although it is rare, there are humans with 4 distinct sets of DNA; in theory, more are possible, but in reality, the more distinct sets of DNA, the less likely that a viable organism is likely to develop.
- We have many examples of ways in which particular types of cells develop into systems that have emergent functionality that is not coded for at the DNA level. Examples inlcude the immune system and the neural system.
Cells don’t merely multiply, regulate, communicate, move, and explore; they also count, sense force and geometry, create form, and even learn. You have never been just a gene or even a set of genes. Instead, you can safely trace your origins back to a first, single cell within your mother’s womb. Once this first cell came into existence, it began to do things that are not written in DNA. As it multiplied, it created a space in which the emerging cells assumed identities and roles, exchanged information, and used their positions relative to each other to build tissues, sculpt organs, and eventually produce a whole organism—you.
ibid., p 7
Part I: The Cell and the Gene
C1: Not in the Genes
Some history:
- Neither finger prints nor retinal patterns are written in the genes.
- In passing, the book says that the pages of Gregory Mendel’s manuscript remained “uncut” (and thus presumably unread) in Charles Darwin’s library. I wonder what the “uncut” refers to in this context — something about the way in which books or manuscripts were published?
- In 1866 Gregor Mendel published (did?) his work on pea genetics, but it did not catch on until it was reproduced, extended and popularized by William Bateson, circa 1905.
- Oswald Avery, studying different strains of pnemonia during the 1920’s, identified DNA as the factor in producing distinctly different strains. Few people paid attention to this, but one that did was James Watson.
Some basic genetics:
- Genes make up only 1-3% of the human genome; this is true across all organisms, although the proportion varies.
- In DNA, sequences of 3 bases (base triplets), each base being 1 of 4 types, code for the 20 amino acids that make up proteins.
- ChatGPT: “DNA does not directly encode lipids or carbohydrates in the same straightforward manner it does proteins. Instead, the enzymes and regulatory proteins involved in the metabolism, synthesis, and modification of lipids and carbohydrates are encoded by DNA.” The same is true for other biochemical constitutents of cells.
- There is a single base triplet (ATG) that indicates the start of a DNA sequence that codes for a protein and three triplets which can signal the end of the protein (TAG, TGA, TAA). This is true for all living plants and animals.
Mutations:
- Some mutations are easy to understand, and some less so. A mutation that prevents the production of an enzyme that catalyzes the breakdown of a substance in the body can be ameliorated by providing the missing enzyme. However other mutations have much more complex effects, causing deficits in the ealier stages of multi-step processes that contribute to the development of an organism.
- Experiments on Drosophilia, and subsequently on other organisms, identified a class of genes called HOX genes that contribute to the pattern of the body (by controlling the expression of DNA).
- Other experiments in Drosophilia showed that if RNA from a gene called eyeless — involved in the construction of the eye — is inserted in a different part of the fly’s body, it will form an eye regardless of where it is.
- Later experiments showed that a human gene that codes for the production of the eye can, if inserted into the chromosome of Drosophilia, result in the production of an eye — but of a Drosophilia eye. The gene says “eye,” but the details of what sort of eye are determined by the cellular context. This is also true for genes that regulate the construction of the nervous system (SOX genes) and the organization of body parts (HOX genes).
Differences across twins:
- A study of 56,000 pairs of identical twins that looked at the occurrence of 560 diseases, found that only 40% appeared to have a strong genetic component.
- Dopplegangers: People who have very similar faces but are genetically unrelated. The implication is that there are some genes that govern the construction of the face that recur, regardless of genetic relationship.
- Identical twins carrying the same genetic mutation may express it very differently. This can be seen by looking at twins and triplets that have heterotaxia (disorganization of body parts, including cleft lips and/or palates).
The chapter ends with an analogy suggesting that DNA is the software, and the celluar system is the hardware, and that the hardware the software runs on is also important/essential.
C2: The Seed of All Things
- Begins with Robert Hooke, who coined the word in Micrgraphia, published in 1665 — arguably the first scientific best seller. He was followed a few years later by van Leeuwenhoek.
- Throughout the 18th and 19th centuries as microscopes and related techniques improved a wide variety of cells were described. There are at least 200 different types of cells in the human body, many with multiple varieties.
- A sketch of a cell: the membrane which contains the cytoplasm and organelles (mitochondria, chloroplasts, centrioles, golgi bodies, ribosomes, xxx) and nucleus, and is structured by the cytoskeleton.
- Cell division: Aided by centrioles which create spindles of filaments and aid mitosis.
- Cell division is determined by many factors including availability of nutrients, volume, composition, stiffness, and signals from its neighbors. A protein called mTOR plays a role in sensing these factors and determining when the cell will divide.
- The number of chromosomes do not seem related the the organisms complexity.
- During creation of gametes, cells divide twice, reducing 46 chromosomes to 23; and during this process, the chromosomes exchange genetic material so that the offspring does not end up with exact copies of its parents chromosomes. {I’m not sure how that works}
- Brief reference to Nick Lane’s Vital Question, in which he suggests cells (as partitions in undersea vents) preceded nucleic acids…
- Life began around 4Ga with prokaryotes, and then eukaryotes (likely a fusion of an archaea and a bacteria) appeared around 2 Ga. Endosymbiosis.
This chapter repeats the litany that for DNA to do anything, it needs a cell. For emergence to happen, things need to interact, and thus they need a space and medium within which to do so.
… reading break …
C3: A Society of Cells
- Evolution of Life: Prokaryotes (4Ga) => eukaryotes (2 Ga) => multicellular organisms (sponge-like) at 0.600 Ga, and the Cambrian explosion at 0.535 Ga, correlated with ‘significant’ (~15%) levels of Oxygen. (Example: Volvox: sphere with cells that have specialized for movement, photoreceptors, and reproduction (sexual or asexual)
- Clonal multicellularity: ability of cells to stay together and differentiate so as to specialize.
- Plants vs. Animals: In plants, cells are like bricks; they get added onto one another, but they don’t move. In animals, cells come in many flavors and move, are pliable and combine with one another in different ways.
- A reference to using genome similarity to judge how closely organisms are related – phylogenetics.
- Choanoflagellates are protists that have an ovoid body like a cap with an extending flagella-tail, giving it a funnel-like appearance. These organisms have many of the same proteins that animals do, including proteins that allow them to stick together to form structures, change their body shape using their cytoskeleton, and surround themselves with an extracellular matrix. At the same time, they lack genes for signaling system (for sensing the state of other cells and their environment) and transcription factors (that allow cell types to be modified into different varieties.
- There is Capaspora, a protist cousin of Choanoflagellate,that have epigenetic machinery for modifying gene expression, and genes that make the protein Brachyury that is the same protein responsible for short tails and spines in mice. When Capaspora’s Brachyury gene is inserted into a frog, it can be used.
- Sponges appear to be the closest relatives of protists on the animal side of the divide. Like plants they are imobile and use choanocytes (that look quite similar to Choanoflagellates) to create currents that bring food to them. Unlike plants, they exhibit cellular diversity, and have the cellular signaling that enables inter-cell communication.
- Cellular diversity. Animal cells have the ability to generate many different varieties of cel, and the cells can stick together in much more organized ways: the can form tubes, sheets, and fibers, and can loosen up and move to a different location and attach to other cells.
- Time and genetic programs. Animal cells (like protists) have lifecycles that unfold over time in irreversible sequences. A gene triggers the production of a transcription factor (a protein) which in turn leads to the expression of a set of genes which sequentially trigger one another leading to materials needed to build a certain structure like a flagellum. These are called genetic programs.
- Inter-cellular signaling for coordination. These genetic programs can run in sequence or in parallel and thus something is required to coordinate these genetic programs. We don’t know what this is in general, but we know that in slime molds cATP is used to signal when individual cells aggregate into a multicellular organism.
- Cellular space and form. Cells also need to create space, both a space they inhabit within an environment, and a sort of intra/intercellular space. Slime molds do this temporarily, but animals do this in a persistent fashion that lasts over long periods of time. This sort of multi-cellular engineering is what is really evident during the Cambrian Explosion of 536 Ma.
- Transcription factors and Cellular Signaling. Genes have transcriptional control regions which are landing pads for transcription factors (protein) and enable the cell to regulate gene expression (or genes to tune their expression depending on what proteins are present in their environment.).
- The Selfish Gene and the Selfless Cell. There was a sort of rebuttal to Dawkin’s “Selfish Gene,” and then a discussion of the selfishness-vs.-selflessness of cells. I don’t find this sort of teleological framing helpful or enlightening.
Part II: The Cell and the Embryo
C4: Rebirths and Ressurections
If the DNA is the instruction manual and the cells the builder, just what is it that the cells do and how do they do it? This chapter takes that off, starting off with embryos and the process of differentiation among cells.
To begin, even a seemingly single type of cell that produces the same organ or tissue undergoes a wealth of genetic activity, exhibiting genetic variations a theme:
Even within a single layer of a single structure, cells exhibit startling variety. A palisade of cells a layer of cells stacked in an organized fashion— spans the length of your digestive tract, but even if we tighten our focus on just this palisade in just this tract, we will witness remarkable variety in cell type and function. Those cells near the beginning of the tract, in the esophagus, are stacked in thick layers to provide a barrier of protection against incoming, undigested food. By the time that food gets to your stomach, this palisade has become a single layer of diverse cell types, some of which secrete acids and enzymes to break down food, while others secrete hormones to regulate the stomach’s activities and defend against bacterial threats. Still lower, a single layer of cells lines the the nutrient particles from digested food, while others secrete a dif. ferent set of hormones, including those that trigger postprandial drowsiness or protect your intestine from acidic juices that might leak from your stomach. The society of cells is not homogenous, and this creates opportunities for cooperation with functional con-sequences.
— Alfonso Martinez Arias, The Master Builder, p. 115-116
Other points from this chapter:
- Totipotency, limited. The zygotę, from which the embryo forms, is totipotent, meaning that it is able to develop into any type of cell in the organism. This totipotence persists, but only for a while, and the degree to which it persists depends on the organism. (This was discovered by Hans Driesch, who working on the embryos of sea urchins. He found that if the cells of a 2- or 4-cell sea urchin blastomere were physically separated each, would develop into a fully functional organism; this ability was lost, though after the zygote had divided further. (
- Fusion and chimeras. It is also the case that if two zygotes are fused, they can develop into a single organism, albeit one with two genomes: a chimera,
- Clonning frogs. Briggs and King succeeded in cloning frogs by inserting a donor nucleus into an oocyte (an unfertilized egg). However, they could only succeed with early cells that had not undergone very many rounds of differentiation.
- Totipotency, restored. However, although totipotence may appear to be lost, it may persist in some cells in the organism. Gurdon replicated Bragg and Kings results, but was able to restore totipotence when he inserted nuclei from the intestines of tadpole frogs, even though those cells were much farther down the cascade of differentiation.
- Clonning Mammals. Clonning mammals is difficult because (1) the mammalian egg is 3 orders of magnitude smaller than a frog egg, and (2) once the donor nucleus is inserted into the egg, it must be implanted in the womb of the mammal…
- Transgenic bacteria. Researchers discovered that they could insert genes for a particular protein (e.g. human insulin) into bacteria, rendering them transgenic, and turn them into hormone factories.
- Limits of bacteria as hormone factories. Bacteria cannot produce all substances, because some of hormones, etc., require subsequent modification which can only take place in the cells of the original organism.
- Transgenic ewes and pharming. It proved possible to modify the mammary cells of a ewe so that she produced Human Factor Ix in her milk. However, such a sheep, might not pass on the ability to its offspring produced by regular sexual reproduction. Instead, this led to an interest in cloning transgenic sheep to produce hormones — this was called “Pharming.”
- Pharming did not turn into a big thing because it is difficult, expensive and takes a long time. There are some niches — breeding cows that produce large milk yields, and racehorses — but most attempts to create a viable business model around pharming have failed. It is also the case that cloning does not necessarily create genetically identical organisms…
- Clonning does not produce genetically identical females. Even clones are not exact genetic replicas, at least in terms of the genes they express.Hence, the case of the clone, copycat was a tabby, but its progenitor was a calico. Female clones, at least, have two copies of the X chromosome, and, on a cell-by-cell basis, the genes one on chromosome or another are suppressed to allow their complements expression. [Is this generally true, or just for females?]
- Clonning to ‘resurrect’ an extinct species is dubious. because there is no oocyte into which insert genetic material. The best that can be done is to try to insert some ancient traits into a non-extinct species.
- Gene expression in cells is controlled by control regions, which can be used to prevent the expression of a particular gene.
- The lac operon. If cells have access to both glucose and lactose, they will first digest all the glucose,m and only when that is gone will they turn to the lactose. The way it works is that two proteins are needed to digest lactose: lactose permease, to transport it into the cell, and B-galactosidase to break it down. Normally, a repressor protein binds to the control regions of these two genes preventing the lactose-digesting proteins from being made. I am confused. One (or both?) of two things happen:
(1) When lactose passively diffuses into the cell, it gets transformed into allolactose which turns off the repressor, enabling production of proteins that enable the absorption and breakdown of lactose. (2) But it is also the case that lactose is not used when glucose is present — One thing I read suggests that production of lactose-digesting proteins only kicks into high gear when glucose is low — low glucose stimulates the production of CAP-cAMP complex which binds to a promoter region leading to production of the lactose-digesting enzymes, figuratively stepping on the accelerator pedal.
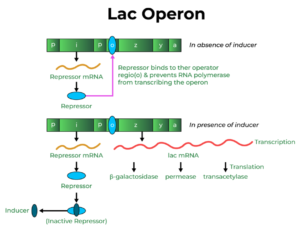
Genes lead to proteins, some of which are transcription factors, that search for control regions to activate other genes, some of which are transcription factors, and so forth. Control regions in A, B, C, D, E, and F determine which genes are activated depending on the sequence of transcription factors. This is how individual circuits become programs. Over the last few years, we have learned that every one of these decisions involves sometimes hundreds of genes that endow each of those cell types with its characteristics.
[…]
[While the above is true for E-Coli, multicellular are more complicated:]
A and B are, more often than not, groups of cells. Yet, even considering the large number of genes involved in every decision, the underlying principle remains the same: cells receive and integrate signals, and then, using special proteins, they seek the control regions of genes that they need to switch on or off, as each activated circuit leads to another, and another.
— Alfonso Martinez Arias, The Master Builder, p. 140
- The Waddington landscape. An allegory involving a ball rolling down a grooved mountain. This is called the epigenetic landscape, meaning that there are factors other than just genes controlling what occurs. This is distinct from the newer meaning of epigenetic which has to do with environmental factors that control gene expression through methylation.
… reading break …
C4: Moving Patterns
This chapter focuses on what happens in the early stages of the development of the embryo — this is the period from the formation of the blastula to the differnetiation of the embryo.
- Early on, the development of the embryo had been observed by carving windows in eggs (Aristotle), or watching development in transparent eggs. It was clear that order emerged from seeming uniformity. There were two schools of thought: one that thought the complete embryo already existed but was too small to see; and the other that argued that cells differentiated into the embryonic structure.
- In the 1828 Karl Ernst von Bayer observed that at an early stage, the embryos of radically differnet organisms appeared quite similar — for example, all animal embryos had gill slits.
- It was not until 1994 (!) that Denis Doboule observed that there was a sort of developmental hourglass: embryos started out distinct, but as they developed went through a bottleneck where they became very similar, before they again began diversifying.
- Doboule noted that the bottleneck corresponded to the time when the HOX genes were fully expressed in a head-to-tail axis in bilateral animals likes frogs and fish.
- The process begins with the fertilization of an egg by a sperm, and the zygote then quickly develops into a mass of undifferentiated cells called the blastula. These typically sit on top of the ‘yolk’ of the egg in a disk, or grow around it to enclose the yolk.
- At a time that is specific for each organism, some epithelial cells transform into the mesenchyme, and mall clusters of these cells began to move around the bastuala. This process is referred to as gastrulation:
By the end of these movements, von Baer’s uncanny structure will be visible — an embryo with a headlike form at one end, a tail at the other, and groups of cells, or segments, running between and within the layers that are starting the process of differentiation into various tissues and organs. This structure is always alike for every animal within each major phylum. We are now at the neck of Duboule’s hourglass, the Hox genes have been unfolded, and the cells have performed their “Hox trot.”
— Alfonso Martinez Arias, .ibid, page 53
- In more detail, what generally happens is that the bastula differentiates into three layers: the endoderm, from which the gut, liver and associated organs will develop; the ectoderm, which will give rise to the skin and brain; and the mesoderm, which will produce the heart, blood, kidneys and genitals.
… reading break …
C6: Hidden from View
This chapter takes its name from fact that unlike fish and birds, mammalian embryos develop inside their mothers, and, in placental mammals, are further enveloped in the placenta
- Mammals comprise about 6,000 distinct species, relative to the 8.5 million species of plants and animals.
- In humans the zygote is not implanted in the uterus until day 7.
- Stages: 0. zygotę => 1. morula (‘berry’) => …primitive endoderm + trophectodern…. => 2. blastocyst
- 1.1. after the zygote divides 3 or 4 times the morula forms a tight cluster of cells — each of which is totipotent.
- 1.2. The morula forms a pallisade of cells that begins to separate cells that will form the embryo from cells that will form the precursor to the placenta (the trophectoderm) and will nourish and protect the embryo.
- 1.3. As the trophectoderm forms, the remaining cells become the primitive endoderm, what will eventually develop into the embryo.
- 1.4. At any point until the formation of the blastocyst is complete, a cell from the trophectoderm can be moved to the primitive endoderm and it will act like a primitive endoderm cell; and vice versa.
- 1.5. The trophectoderm will use a protein called CDX2 to control its structure and trigger the creation of a collection of pumps that when turned on will introduce fluid and create the beginnings of a yolk sac of 100-150 cells.
- 1.6. More generally, throughout stage 1 (and maybe later?) the cells are using FGF, Wnt and Nodal proteins for signaling, and a new signaling system called Hippo which conveys information about the number and position of cells).
- 2. Blastocyst: embryo + primitive { gut | endoderm} (yolk sack)
- The mother and embryo can exchange cells, and in particular the mother can receive multi-potent cells that she can use to repair damaged tissues, including those in her own body. This is known as fetal cell microchimerism.
- Much progress was made by collecting and studying morula, blastocysts, and embryos obtained from miscarriages by methods that would today be questioned. Donated embryos, etc., were attributed to the doctors, et al., who gathered them, and not to the mothers who produced them and likely had little/no knowledge of what was going on.
- The majority of in vivo pregnancies are non-viable: many morula fail to implant, and 10-20% more implanted blastocysts and embryos are non-viable. In vitro fertilization has a similar success rate.
- In 1982 the UK convened a committed headed by Mary Warnock, a philosopher, to establish guidelines on the ethical use of early-stage embryos. They ended up deciding there was a clear line at 14 days, the point where cell in the embryo stopped being totipotent and the primitive streak occurred, and splitting an embryo would no longer produce identical (or even viable) embryos.
- During development of the embryo, cell division occurs at an enormous rate: neural cells are created at the rate of 10,000/minute.
- Mutations occur frequently, even when a cell is not dividing, and they occur at a fairly constant rate. A neural cell contains approximately 1,500 mutations. Most mutations have no effect because (1) only 2% of genes code for growth, and (2) we mostly have two chromosomes so there are redundant genes, and (3) most of the mutations mentioned are only in a few cells.
- It is not likely that any human has two cells with identical genomes. Mutations occur frequently, even without division, and more frequently with division. We are genetic mosaics.
Every cell division in human somatic tissues has about 1/10th chance of a mutation event. (Per base mutation rate 1×10-10, diploid genome size 6.4×109 bases, 6.4 billion bases. Each cell of your body undergoes between 50 and 3000 cell divisions in a lifespan, by our estimates. You have trillions of cell divisions in your life. Your genome is riddled with mutations. Likely every single base in your genome has been mutated at least once in your body.
90% of mutations are harmless because our genome largely consists of transposon expansion, the corpses of no longer functional selfish genetic elements. 1% is coding, and scientists generally generously estimate the regulatory genome to be perhaps 9%, also it makes for easier calculations. The worse mutations are cellular lethals and create nonfunctional cells– and are selected out of the extant cell population. The exact distribution of dna mutation across our bodies is unknown, no one has sequenced quite that much.
Helmdacil
… reading break …
C7: Renewal
- The Immortal Chicken, NOT. Although Alexis Carrell claimed to be able to keep chicken cells reproducing in a culture for 34 years, no one else was able to reproduce his results.
- The Hayflick limit. In 1961 Leonard Hayflick challenged Carrell’s results, showing that normal cells could only reproduce about 50 times — this is called the Hayflick limit.
- Zombie DNA. DNA remains active in an organism for up to 2 days after death.
- Immortal cancer cells and HeLa. While normal cells undergo sentence, cancer cells do not. They are effectively immortal. This is the case with what are now called HeLa cells, taken from a cervical tumor in Henrietta Lacks. At the time, the collection/culturing of tumor cells (without premission or informed consent) was a standard practice and performed on all cancer patients (at least at this institution). The researcher who collected the HeLa cells shared them with other researchers, and they became a sort of standard of research. (These are not the only lines of ‘immortal cells — other lines of immortal cells have also been found.) HeLa cells are not normal cells: they have anywhere from 35-45 pairs of chromosomes (vs. 23 in a normal human), and over the decades have undergone enough mutation that it is difficult to call them human, let alone Henrietta Lacks’ cells. Various versions of HeLa cells also differ considerably from one another).
- Telomeres and telomerase. On the ends of a chromosome is a pattern of bases called a telomere. This normally shortens as the cell divides. Cancer cells, however, have long telomeres, and also very high levels of an enzyme called telomerase which protects the telomeres. Adding telomerase to cells in a normal culture will allow them to continue dividing beyond their Hayflick limit, but the cell’s still age (i.e. undergo mutations).
- Adult stem cells. In humans, various tissue cells divide at various rates. The body produces a new intestinal lining about once a week; skin and blood is also produced at high rates. This turnover is due to the work of “adult stem cells” which age very slowly and produce new cells. Most tissues and organs have their own adult stems cells — the brain, which was thought to be an exception, appears to have stem cells for the dentate gyrus area in the hippocampus, associated with learning and memory.
- The Niche. Adult stem cells are typically found in a protected area of their organ or tissue called the niche. Outside of their niche, stems cells transform into normal cells, and age accordingly.
- Miniguts and Organoids. Toshiro Sato succeeded in harvesting mouse intestinal stem cells and culturing them in an environment that resembled that of their niche. After a few days, the stem cells did something surprising: they formed spikey hollow cells built out normal intestinal cells with stem cells regularly spaced at their bottoms. After a few days these evolved into “miniguts,” miniature simplified versions of the intestine which could reproduce themselves in the culture. These are the first, but not the only, example of organoids.
- Other organoids include mini-livers, ,mini-lungs, mini-pancreases, mini-brains and organoid skin. In all cases Wnt signaling and Madrigal appear to be essential to creating organoids.
- Organoids are important because, while they do not function like the actual organs, they offer a way of studying the effects of various treatments on the tissue in question.
- Tetranomas and Embryo Carcinoma Cells. Leroy Stevens discovered the mouse tetranomas, when injected into the intestinal lining, generated clusters of cells that looked like early embryos. He also discovered that injecting cells from early embryos into the testes would give rise to tetranomas. He called these cells embryo carcinoma cells.
- Embryonic Stem Cells. Other researches discovered that cells taken from a mouse blastomere before it was implanted in the uterus could be injected into other mouse embryos and would be incorporated and would produce fertile mice.
- Human embryonic stem cells. In 1998 human embryonic stem cells were isolated and verified. This was important because if researchers could learn how to direct them, they might be used to regenerate nerve cells or other tissues. These cells appear to maintain their pluripotent state by saturating themselves in a cocktail of signals familiar from gastrulation when cells begin to take their final position in the body plan: FGF, Nodal and Wnt.
- Induced pluripotent stem cells (iPSC). Shinya Yamanaka discovered how to transform normal adult human cells into human embryonic stem cells. This involved using the genes for the transcription factors: Sox2, Klf, Oct4 and Myc. First he figured it out for mice; then he applied it to human cells. He calls the resulting cells induced pluripotent stem cells (iPSC).
- The Imaginal disk. In a fruit fly maggot, at the time of gastrulation, small groups of cells called imaginal disks are set aside in various regions of the fruit fly embryo. When the maggot hatches out of the egg, the imaginal disks grow, along with other cells. When the maggot forms a pupae or chrysalid, the maggot cells die, and the imaginal disks come together and assemble the insect. In essence, a maggot is sort of a mobile, nutrition-seeking egg.
… reading break …
C8: The Embryo Redux
- Recap on Fruitflies: They share 75% of human genes, and sleep, get depressed, court and count. They have a been a major vehicle for carrying out genetic research. But they develop so quickly, that it is difficult to intervene. Research mostly involves mutating genes, and see what transpires. But one cannot — in tune with Feynman’s dictum ‘What I cannot create, I cannot understand’ – intervene in the rapid development process.
- Around 2000, Arias and his lab began experimenting with embryonic stem cells from mice. They observed that such cells, when placed in an environment that challenged the cell to make mesoderm or endoderm, that the cells would follow the trajectories and schedule of development appropriate to their environment.
- Upon learning that Sato and Clevers had discovered how to create intestinal organoids by allowing stem cells to develop in an environment that permitted 3D growth, and then upon learning about how carcinoma cells could generate a structure similar to that of an early embryo, they tried a similar experiment using mouse embryonic stem cells. As the cells developed, the filmed them, and realized the cells were trying to gastrulate… eventually, they saw that they were creating gastruloids.
- “In essence, all organoids are disembodied sketches of our organs.” p. 253
- As they explored gastruloids, they were able to see how the cells self-organized and created their own coordinate systems. For years scientists had been aggregating embryonic stems cells into structures called embryoids and observing chaotic specialization. What about gastruloids was leading to the self organization? They saw two factors: one was the signaling regime, starting with Ant; the other was the initial number of cells present. The magic number was around 400: too few, and nothing happened; too many and mishapened and confused structures resulted. With around 400 (about the same number as when gastrulation occurs in situ), gastruloids would form, and with about 450, twin gastruloids would form.
- Other researchers, by adding extra embryonic cells (that contribute to the placenta and the yolk sac), have gotten the stem cells to form clusters that strongly resembler embryos. Arias finds this interesting, but thinks that gastruloids offer a better pathway for learning more about self-organization.
- Gastruloids offer evidence that clusters of stem cells have the ability to take their cues from one another and from their environment.
- After producing mouse gastruloids, Arais’ lab also was able to produce human gastruloids.
- Duboles’s hourglass: Early on embryos of different organisms look different because they are developing from, and thus constrained by, very different egg architectures. Later on embryos of different organisms look different because they are developing into different organisms. But there is a period in between, when all the embryo’s look very similar, just when the Hox genes are expressed — the idea is that at this point the embryo is constrained to use the tools in Hox genes to build the body plan.
Taken together, these experiments suggest that by releasing embryonic stem cells from the constraints of the egg, as we do in our gastruloid and pescoid experiments, we get to see the cells assemble into a shape that is different from what nature would expect.
Imagine taking a long balloon and twisting it into any number of animal shapes; you can accomplish this through force constraints, but when you remove those forces, the balloon will relax back into its natural form. So it is with cells in an embryo. Further, it appears that gastrulation is the period in development when the force constraints of the egg are removed. Somewhat unexpectedly, the cells, regardless of their animal of origin, produce the same shape, a simple, polarized structure with Brachyury expressed at one end, the rudimentary heart at the other, a midline, and two sides — in other words, the shape of a gastruloid.
These experiments revealed an underlying common pattern, a basic ground plan, clay for sculpting animals. Whatever genes do in the development of an organism, the evolution of life-forms is constructed by the geometry and mechanics of cellular ensembles. Whether generated from fish, frog, mouse, or human cells, when freed from the constraints of the egg, cells build the same simple shape—a basic and conserved form that we have called the morphogenetic ground plan. Gastruloids are one incarnation of this plan, which I believe is common to all animals and probably originated in the days of the Burgess shale, when there was a huge explosion in animal diversity, with cells-not genes-trying out different constructions. As noted in Chapter 3, the defining event of life on earth, the emergence of animals from unicellular ancestors, rests on cells’ discovery that they can work in ensembles to conquer and sculpt space.
— ibid., p 263
C9: On the Nature of a Human
This chapter discusses with these *-oids can be thought of as having similar statuses to the entities they resembler, or whether they are different.
ChatGPT on Blastoids and Gastruloids
Blastoids and gastruloids are both stem-cell-derived structures that model early stages of embryonic development, but they represent different phases and serve distinct research purposes.
Blastoids are artificial structures that resemble the blastocyst stage of an embryo, which occurs about five to six days after fertilization in humans. A natural blastocyst consists of an inner cell mass (future embryo) and an outer layer called the trophectoderm (future placenta). Blastoids are created from pluripotent stem cells and mimic the spatial organization, gene expression, and functionality of natural blastocysts. They are valuable for studying early embryonic development, implantation processes, and can help in understanding infertility and developmental defects without using actual embryos.
Gastruloids, on the other hand, model the gastrulation stage, which follows the blastocyst stage and occurs after implantation. Gastrulation is a critical phase where the embryo begins to establish the three primary germ layers: ectoderm, mesoderm, and endoderm. Gastruloids are generated from embryonic stem cells and replicate aspects of early embryonic patterning, symmetry breaking, and the formation of the body axis. They are instrumental in studying how cells differentiate and organize into complex tissues and organs.
The relationship between blastoids and gastruloids lies in their sequential representation of embryonic development stages:
- Blastoids model the pre-implantation blastocyst stage.
- Gastruloids model the post-implantation gastrulation stage.
Both serve as ethical and practical alternatives to using actual embryos, allowing researchers to investigate fundamental developmental processes in vitro. By providing insights into different stages of embryogenesis, they collectively enhance our understanding of human development, congenital disorders, and potential regenerative medicine applications.
# # #
Views: 16